- 2023 Lancet Countdown U.S. Launch Event
- 2023 Lancet Countdown U.S. Brief
- PAST BRIEFS

2020 CASE STUDY 2
The 2019 floods in the central u.s..
Lessons for Improving Health, Health Equity, and Resiliency
In spring 2019, the Midwest region endured historic flooding that caused widespread damage to millions of acres of farmland, killing livestock, inundating cities, and destroying infrastructure. CS_52
The Missouri River and North Central Flood resulted in over $10.9 billion of economic loss in the region, making it the costliest inland flood event in U.S. history. CS_52 Yet, this is just the beginning, as climate change continues to accelerate extreme precipitation, increasing the likelihood of severe events previously thought of as “once in 100 year floods.” CS_53 , CS_54
This 2019 disaster exhibited the same health harms and healthcare system disruptions seen in previous flooding events, and vulnerable populations – notably tribal and Indigenous communities – were once again disproportionately impacted. Thus, there is an enormous need for policy interventions to minimize health harms, improve health equity, and ensure community resilience as the frequency of these weather events increases.
Before-and-after images of catastrophic flooding in Nebraska. Left image taken March 20, 2018. Right image taken March 16, 2019.
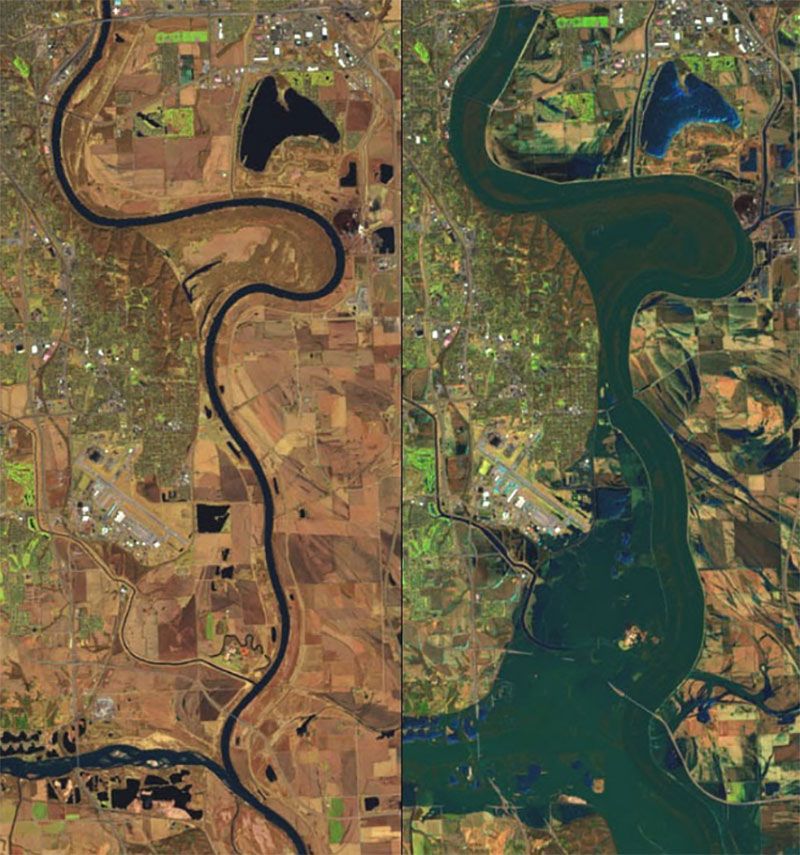
Source: NASA Goddard Space Flight Center, with permission
The role of climate change, widespread devastation, and compounding inequities
The Missouri River and North Central Flood were the result of a powerful storm that occurred near the end of the wettest 12-month period on record in the U.S. (May 2018 – May 2019). CS_55 , CS_56 The storm struck numerous states, specifically Nebraska (see Figure 1), Iowa, Missouri, South Dakota, North Dakota, Minnesota, Wisconsin, and Michigan. Two additional severe flooding events occurred in 2019 in states further south, involving the Mississippi and Arkansas Rivers.
This flood event exhibits two key phenomena that have been observed over the last 50 years as a result of climate change: annual rainfall rates and extreme precipitation have increased across the country. CS_57 The greatest increases have been seen in the Midwest and Northeast, and these trends are expected to continue over the next century. Future climate projections also indicate that winter precipitation will increase over this region, CS_57 further increasing the likelihood of more frequent and more severe floods. For example, by mid-century the intensity of extreme precipitation events could increase by 40% across southern Wisconsin. CS_58 While it is too early to have detection and attribution studies for these floods, climate change has been linked to previous extreme precipitation and flood events. CS_59 , CS_60
Hundreds of people were displaced from their homes and millions of acres of agricultural land were inundated with floodwaters, killing thousands of livestock and preventing crop planting. CS_52 , CS_61 , CS_62 Federal Emergency Management Agency (FEMA) disaster declarations were made throughout the region, allowing individuals to apply for financial and housing assistance, though remaining at the same housing site continues to place them at risk of future flood events.
In Nebraska alone, 104 cities, 81 counties and 5 tribal nations received state or federal disaster declarations. FEMA approved over 3,000 individual assistance applications in Nebraska, with more than $27 million approved in FEMA Individual and Household Program dollars. In addition to personal property, infrastructure was heavily affected, with multiple bridges, dams, levees, and roads sustaining major damage (see Figure 2). CS_52
Destruction of Spencer Dam during Missouri River and North Central Floods. CS_63
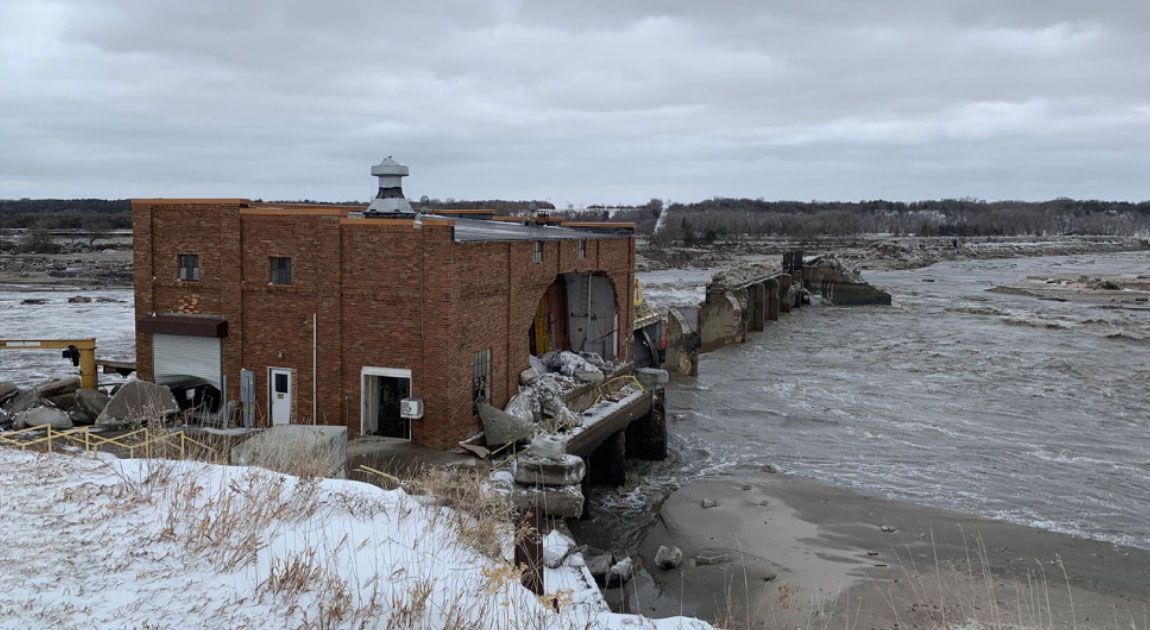
- Oglala Sioux Tribe, Cheyenne River Sioux Tribe of the Cheyenne River Reservation, Standing Rock Sioux Tribe (North Dakota and South Dakota), Yankton Sioux Tribe of South Dakota, Lower Brule Sioux Tribe of the Lower Brule Reservation, Crow Creek Sioux Tribe of Crow Creek Reservation, Sisseton-Wahpeton Oyate of the Lake Traverse Reservation, Rosebud Sioux Tribe of the Rosebud Sioux Indian Reservation, Santee Sioux Nation, Omaha Tribe of Nebraska, Winnebago Tribe of Nebraska, Ponca Tribe of Nebraska, Sac & Fox Nation of Missouri (Kansas and Nebraska), Iowa Tribe of Kansas and Nebraska, and Sac & Fox Tribe of the Mississippi in Iowa.
Source: Nebraska Department of Natural Resources, with permission.
As with other climate-related disasters, the 2019 floods had devastating effects on already vulnerable communities as numerous tribes and Indigenous peoples were impacted,° adding to centuries of historical trauma. CS_64 , CS_65 Accounts of flooding on the Pine Ridge Reservation in South Dakota demonstrate the challenges that resource-limited communities face in coping with extreme weather events. CS_64 Delayed response by outside emergency services left tribal volunteers struggling to help residents stranded across large distances without access to supplies, drinking water, or medical care.66 Lack of equipment and limited transportation hampered evacuations. CS_67
Health harms and healthcare disruptions
There were three recorded deaths from drowning, but hidden health impacts were widespread and extended well beyond the immediate risks and injuries from floodwaters. In the aftermath, individuals in flooded areas were exposed to hazards like chemicals, electrical shocks, and debris. CS_68 Water, an essential foundation for health, was contaminated as towns’ wells and other drinking water sources were compromised. This put people, especially children, at increased risk for health harms like gastrointestinal illnesses. CS_69 Stranded residents relied on shipments of water from emergency services and volunteer organizations and the kindness of strangers ( see Box 1 ).
BOX 1: “We just remember the trust and commitment to each other”
Linda Emanuel, a registered nurse and farmer living in the hard-hit rural area of North Bend in Nebraska, helped organize flood recovery efforts. She recalled wondering, “How are we going to handle this? How do we inform the people of all the hazards without scaring them?” In addition to her educational role, she administered a limited supply of tetanus shots, obtained and distributed hard-to-find water testing kits, and coordinated PPE usage. In the first days of the flooding, she hosted some 25 stranded individuals in her home. Reminiscing about how community members came together amidst the devastation, Emanuel remarked, “We just remember the trust and the commitment to each other and to our town. We are definitely a resilient city.” CS_70
Standing water remained in many small town for months, and a four-year old child at the Yankton Sioux reservation in South Dakota likely contracted Methicillin-resistant Staphylococcus aureus (MRSA) after playing in a pond. CS_71 The mold and allergens that developed in the aftermath of the floods exacerbated respiratory illness. CS_72 Flooding also backed up sewer systems into basements; clean up required personal protective equipment (PPE) to prevent the potential spread of infectious diseases. The significant financial burdens, notably the loss of property in the absence of adequate insurance, can contribute to serious mental and emotional distress in flood victims. CS_73 , CS_74
Infrastructure disruptions, like flooded roads, meant that many individuals in rural areas were unable to access essential services including healthcare. In an interview with the New York Times, Ella Red Cloud-Yellow Horse, 59, from Pine Ridge Indian Reservation, recounts her own struggle to get to the hospital for a chemotherapy appointment. CS_64 After being stranded by flooding for days, she had contracted pneumonia, but she couldn’t be reached by an ambulance or tractor because her driveway was blocked by huge amounts of mud. She was forced to trudge through muddy flood waters for over an hour to get to the highway.
She told the Times, “I couldn’t breathe, but I knew I needed to get to the hospital.” Her story is an increasingly common occurrence as critical infrastructure is damaged by climate change-intensified extreme events. These infrastructure challenges are also often superimposed on top of the challenges of poverty and disproportionate rates of chronic diseases ( see the Case Study ). Multiple hospitals sustained damage and several long-term care facilities were forced to evacuate, with some closing permanently, as a result of the rising floodwaters, CS_75 likely exacerbating existing diseases.
A path towards a healthier, equitable, and more resilient future
As human-caused climate change increases the likelihood of precipitation events that can cause severe flooding disasters, public health systems must serve as a first line of defense against the resulting health harms. As such, the broader public health system needs to develop the capacity and capability to understand and address the health hazards associated with climate-related disasters. Often funds and resources for these efforts are focused on coastal communities; however, inland states face many climate-related hazards that are regularly overlooked. Building on or expanding programs similar to CDC’s Climate-Ready States and Cities Initiative will help communities in inland states prepare for future climate threats. CS_76
Additionally, public health officials, health systems, and climate scientists should collaborate to create robust early warning systems to help individuals and communities prepare for flood events. Education regarding the health impacts of flooding should not be limited to the communities affected, but it should also include policymakers and other stakeholders who can implement systemic changes to decrease and mitigate the effects of floods. Local knowledge offered by community members regarding water systems, weather patterns, and infrastructure will be essential for effective and context-specific adaptation. By implementing these changes and executing more inclusive flood emergency plans, communities will be better situated to face the flood events that are projected to increase in the years to come.
Introduction – Figure 1: Nebraska Flooding The Role of Climate Change – Figure 2: Destruction of Spencer Dam Health Harms and Healthcare Disruptions – Box 1: Remember the Trust A Path Towards Equality
3.16 Case Study - Flooding in Somerset (2013-2014)
For a period of three months from December 2013 to February 2014, the Somerset Levels hit the national (United Kingdom) headlines as the area suffered from extensive flooding. At the height of the winter floods, 65 km2 of land on the Levels were under water. This was caused by human and physical factors. The floods were the most severe ever known in this area.
No one was prepared for the extent of damage brought by the floodwater. Several villages and farms were flooded and hundreds of people had to be evacuated. The risk of flooding is likely to increase in the future due to climate change. The government will need to invest in flood defences in order to protect areas at risk.
Flooding on the Somerset Levels - The News
Flooding on the Somerset Levels - Background Information
In December 2013, an unusually high amount of rainfall began to fall on the Somerset Levels and this continued into February 2014. With so much water, the ground became saturated, forcing both the river Parrett and the river Tone to flood.
The physical characteristics of the Somerset Levels and Moors mean that flooding is a natural occurrence there. It is an area of low-lying farmland and wetlands between the Mendip and Blackdown Hills in central Somerset. This area forms the floodplain surrounding the river Parrett.
Thousands of years ago the area was covered by the sea. It has since been drained to allow for agriculture, several villages and wetland conservation. It has become an area of social, economic and environmental importance. It covers an area of 650 km2 but has a low population density (the number of people per km2 ). The area may have had a natural vulnerability to floods but no one was prepared for the scale of the floods or the impacts that followed
What caused the flooding on the Somerset Levels?
A river flood is when the river bursts its banks and spills onto the surrounding floodplain. A floodplain is an area of low-lying ground next to a river, formed mainly of river sediments. A flood can last just a few days or several weeks. A flood event is often caused by a combination of physical and human factors.
Physical causes
Prolonged rainfall: In January 2014 in southern England, rainfall totalled 183.8 mm, which is approximately 200% higher than average for that month (Figure 2). That was the wettest since records began in 1910.
Saturated ground: The long period of rainfall caused the ground to become saturated so that it could not hold any more water.
Low-lying land: Much of the area lies at, or just a few metres above, sea level, putting it at risk of flooding.
High tides and storm surges from the Bristol Channel: These prevent the floodwater from being taken to the sea, forcing it to back up the rivers.
Human Causes
Lack of dredging: Over the years the rivers had become clogged with sediment. The Environment Agency had decided to stop dredging the rivers some time earlier. Dredging increases the ability of a river to carry more water.
Change in farming practices: Much of the land has been converted from grassland to grow maize. This more intensive use of the land means it is less able to retain water, causing it to run over the surface rather than being absorbed.
Impacts of the Somerset floods
The widespread flooding on the Somerset Levels made the national headlines. Many people visited the affected areas to see the famous floods. Such people became known as ‘flood tourists’. Many of the people living on the Levels had experienced some form of flooding in the past but no one was quite prepared for the scale of these floods. Thankfully no one died, but many people suffered flood damage to their homes, possessions and farmland (Figure 3).
Many people were evacuated and had to seek temporary accommodation elsewhere. More than 600 homes and 6880 hectares of farmland were flooded. Entire villages were cut off after roads became unusable. In the village of Muchelney, residents could only leave the island by a boat which left every two hours (Figure 4).
Isolated communities provided an opportunity for thieves. In January, 900 litres of fuel was stolen from a pumping station in Westonzoyland. By early February, there were reports of stolen heating oil and quad bikes from homes of flood victims. Many of the main roads were closed, such as the A361 which links Taunton and Street. Trains on the Bristol line between Bridgwater and Taunton were also disrupted. The economic costs soon started to rise. Fuel for emergency pumps used to reduce water levels cost £200 000 per week. Local businesses reported over £1 million in lost business. According to ‘Visit Somerset’ the floods on the Somerset Levels cost the county’s tourism industry £200 million.
Farmers struggled to deal with flooded fields, ruined crops and the costs of moving livestock away from the affected areas. After nearly three months under millions of tonnes of water, much of the soil was damaged. It may take up to two years to restore the soil so that crops can be grown. Flood-hit home owners are likely to see their insurance costs increase in the future.
Management and Response
The response to the floods was rapid and well organised, as expected for an economically developed country (Figure 5). The Met Office issued an amber warning for heavy rain in South West England. They informed the public to be prepared for significant flooding. Many residents used sandbags to protect their homes and moved valuable items upstairs. One man even built a giant wall out of clay and soil around his house in Moorland to protect it from the floodwaters.
The fire brigade visited hundreds of properties, and rescue boats were used to help stranded people. In early February, rescue crews encouraged the residents of Moorland to evacuate. Owners of around 80 homes agreed but about 30 other residents chose to remain (Figure 6). Extra police patrols were brought in to respond to increased crime. By the end of January, the army had been sent in with specialist equipment. They delivered food and gave out sandbags. By 6 February they were joined by 40 Royal Marines. Sixty-five pumps were used to drain 65 million m3 of floodwater.
There was a lot of local support for those affected by the floods, led by the organisation FLAG (Flooding on the Levels Action Group). Volunteers organised fundraising activities and collected and distributed supplies of food. They also used social media via Facebook and Twitter to communicate news.
The longer-term response focused on flood management to prevent a future flood of this scale. This took the form of ‘The Somerset Levels and Moors Flood Action Plan’. It included measures such as dredging, a tidal barrage, and extra permanent pumping sites, with a total cost of £100 million. A sum of £10 million was provided by the Conservative Government, a further £10 million came from the Department for Transport, and the Department for Communities and Local Government gave £500 000. It formed part of a 20-year plan for the Levels. It had the backing of Prime Minister David Cameron who stated: ‘We cannot let this happen again’.
Future Considerations
In November 2014, the Environment Agency (EA) kept its promise and completed the 8 km dredging of the rivers Parrett and Tone, costing £6 million. This will be a huge help in the protection of homes and farmland. Some people have argued that dredging alone is not the answer and it should be used alongside other forms of flood defence, such as flood relief channels. Can the government afford to spend so much money in a rural area with a low population?
Climate change may mean that this area will receive more heavy rain in the future. The Met Office has predicted that sea levels around the UK will rise by 11–16 cm by 2030. It may be that spending money on hard engineering flood defences is not the best option for this area. The government may save money in the long term by moving people to higher land, and to pay them money for their homes and farms. However, this is unlikely to be a popular option.
Conclusion
The recent floods demonstrate how more people have put themselves at risk of flooding by living on this low-lying floodplain. Farming and settlement increased because people thought that flooding in the area was under control. This was clearly not the case and it is therefore not surprising that the local people felt so let down. There were many impacts of this flood, but they could have been far worse if it had not been for the effective and rapid response that followed.
Further Information
Click here to return to the Home page.
Thank you for visiting nature.com. You are using a browser version with limited support for CSS. To obtain the best experience, we recommend you use a more up to date browser (or turn off compatibility mode in Internet Explorer). In the meantime, to ensure continued support, we are displaying the site without styles and JavaScript.
- View all journals
- Explore content
- About the journal
- Publish with us
- Sign up for alerts
- Review Article
- Published: 10 August 2021
Causes, impacts and patterns of disastrous river floods
- Bruno Merz ORCID: orcid.org/0000-0002-5992-1440 1 , 2 ,
- Günter Blöschl 3 ,
- Sergiy Vorogushyn ORCID: orcid.org/0000-0003-4639-7982 1 ,
- Francesco Dottori ORCID: orcid.org/0000-0002-1388-3303 4 ,
- Jeroen C. J. H. Aerts ORCID: orcid.org/0000-0002-2162-5814 5 , 6 ,
- Paul Bates 7 ,
- Miriam Bertola ORCID: orcid.org/0000-0002-5283-0386 3 ,
- Matthias Kemter 1 , 2 , 8 ,
- Heidi Kreibich 1 ,
- Upmanu Lall ORCID: orcid.org/0000-0003-0529-8128 9 &
- Elena Macdonald ORCID: orcid.org/0000-0003-0198-6556 1
Nature Reviews Earth & Environment volume 2 , pages 592–609 ( 2021 ) Cite this article
16k Accesses
238 Citations
351 Altmetric
Metrics details
- Natural hazards
Disastrous floods have caused millions of fatalities in the twentieth century, tens of billions of dollars of direct economic loss each year and serious disruption to global trade. In this Review, we provide a synthesis of the atmospheric, land surface and socio-economic processes that produce river floods with disastrous consequences. Disastrous floods have often been caused by processes fundamentally different from those of non-disastrous floods, such as unusual but recurring atmospheric circulation patterns or failures of flood defences, which lead to high levels of damage because they are unexpected both by citizens and by flood managers. Past trends in economic flood impacts show widespread increases, mostly driven by economic and population growth. However, the number of fatalities and people affected has decreased since the mid-1990s because of risk reduction measures, such as improved risk awareness and structural flood defences. Disastrous flooding is projected to increase in many regions, particularly in Asia and Africa, owing to climate and socio-economic changes, although substantial uncertainties remain. Assessing the risk of disastrous river floods requires a deeper understanding of their distinct causes. Transdisciplinary research is needed to understand the potential for surprise in flood risk systems better and to operationalize risk management concepts that account for limited knowledge and unexpected developments.
The causative mechanisms of floods with disastrous consequences tend to be different from those of non-disastrous floods, and show anomalies in one or several flood- and loss-generating processes.
Past trends in flood hazard show both upward and downward changes. In some regions, anthropogenic warming is already strong enough to override other drivers of change.
Flood hazards and impacts are projected to increase for many regions around the globe. Future flooding hotspots are expected in Asia and Africa, owing to climate and socio-economic changes.
Reducing vulnerability is a particularly effective way of reducing flood impacts. Global decreases in flood-affected people and fatalities since the mid-1990s (despite a growing population) are signs of effective risk reduction.
Disastrous floods often come as a surprise. Effective risk reduction requires an understanding of the causative processes that make these events distinct and to address the sources of surprise, including cognitive biases.
This is a preview of subscription content, access via your institution
Access options
Access Nature and 54 other Nature Portfolio journals
Get Nature+, our best-value online-access subscription
24,99 € / 30 days
cancel any time
Subscribe to this journal
Receive 12 digital issues and online access to articles
111,21 € per year
only 9,27 € per issue
Buy this article
- Purchase on SpringerLink
- Instant access to full article PDF
Prices may be subject to local taxes which are calculated during checkout
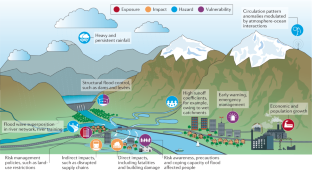
Similar content being viewed by others
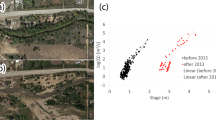
Floods and rivers: a circular causality perspective
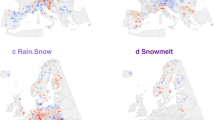
Shifts in flood generation processes exacerbate regional flood anomalies in Europe
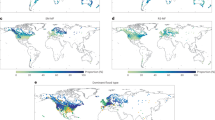
Reconciling disagreement on global river flood changes in a warming climate
Data availability.
The authors declare that the data supporting the findings of this study are available within the article and its supplementary information files. Other data can be provided by the authors on request.
Doocy, S., Daniels, A., Murray, S. & Kirsch, T. D. The human impact of floods: a historical review of events 1980−2009 and systematic review. PLOS Curr . https://doi.org/10.1371/currents.dis.f4deb457904936b07c09daa98ee8171a (2013).
United Nations Office for Disaster Risk Reduction GAR2015 — Global Assessment Report on Disaster Risk Reduction . (UNDRR, 2015).
Jongman, B., Ward, P. J. & Aerts, J. C. J. H. Global exposure to river and coastal flooding: long term trends and changes. Glob. Environ. Change 22 , 823–835 (2012).
Article Google Scholar
Dottori, F. et al. Increased human and economic losses from river flooding with anthropogenic warming. Nat. Clim. Change 8 , 781–786 (2018).
Barendrecht, M. H., Viglione, A. & Blöschl, G. A dynamic framework for flood risk. Water Secur. 1 , 3–11 (2017).
Vorogushyn, S. et al. Evolutionary leap in large-scale flood risk assessment needed. Wiley Interdisc. Rev. Water 5 , e1266 (2018).
Di Baldassarre, G. et al. Debates — perspectives on socio-hydrology: capturing feedbacks between physical and social processes. Water Resour. Res. 51 , 4770–4781 (2015).
Merz, B. et al. Floods and climate: emerging perspectives for flood risk assessment and management. Natural Haz. Earth Syst. Sci. 14 , 1921–1942 (2014).
Merz, B., Hall, J., Disse, M. & Schumann, A. Fluvial flood risk management in a changing world. Natural Haz. Earth Syst. Sci. 10 , 509–527 (2010a).
Chen, Y. et al. Socio-economic impacts on flooding: a 4000-year history of the Yellow River, China. Ambio 41 , 682–698 (2012).
Munoz, S. E. et al. Climatic control of Mississippi River flood hazard amplified by river engineering. Nature 556 , 95–98 (2018).
Blöschl, G. et al. Increasing river floods: fiction or reality? Wiley Interdisc. Rev. Water 2 , 329–344 (2015).
Sharma, A., Wasko, C. & Lettenmaier, D. P. If precipitation extremes are increasing, why aren’t floods? Water Resour. Res. 54 , 8545–8551 (2018).
Ho, M. et al. The future role of dams in the United States of America. Water Resour. Res. 53 , 982–998 (2017).
Kreibich, H. et al. Adaptation to flood risk: results of international paired flood event studies. Earth’s Future 5 , 953–965 (2017).
Lempérière, F. Dams and floods. Engineering 3 , 144–149 (2017).
Kundzewicz, Z. W. et al. Differences in flood hazard projections in Europe — their causes and consequences for decision making. Hydrol. Sci. J. 62 , 1–14 (2017).
Google Scholar
Tarasova, L. et al. Causative classification of river flood events. Wiley Interdisc. Rev. Water 6 , e1353 (2019).
Smith, J. A., Cox, A. A., Baeck, M. L., Yang, L. & Bates, P. Strange floods: the upper tail of flood peaks in the United States. Water Resour. Res. 54 , 6510–6542 (2018).
Rosbjerg, D. et al. (eds.) In Runoff Prediction in Ungauged Basins 189−226 (Cambridge Univ. Press, 2013).
Villarini, G. & Smith, J. A. Flood peak distributions for the eastern United States. Water Resour. Res . 46 , https://doi.org/10.1029/2009wr008395 (2010).
Berghuijs, W. R., Woods, R. A., Hutton, C. J. & Sivapalan, M. Dominant flood generating mechanisms across the United States. Geophys. Res. Lett. 43 , 4382–4390 (2016).
Villarini, G. On the seasonality of flooding across the continental United States. Adv. Water Resour. 87 , 80–91 (2016).
Blöschl, G. et al. Changing climate shifts timing of European floods. Science 357 , 588–590 (2017).
Merz, R. & Blöschl, G. A process typology of regional floods. Water Resour. Res . 39 , https://doi.org/10.1029/2002wr001952 (2003).
Hirschboeck, K. K. Flood hydroclimatology. In Flood Geomorphology (eds Baker, V. R., Kockel, R. C. & Patton, P. C.) 27–49 (John Wiley & Sons, 1988).
Nakamura, J., Lall, U., Kushnir, Y., Robertson, A. W. & Seager, R. Dynamical structure of extreme floods in the U.S. Midwest and the United Kingdom. J. Hydrometeorol. 14 , 485–504 (2013).
Teegavarapu, R. Extreme precipitation and floods. In Floods in a Changing Climate: Extreme Precipitation International Hydrology Series 115−147 (Cambridge Univ. Press, 2012).
McGregor, G. R. Climate and rivers. River Res. Appl. 35 , 1119–1140 (2019).
Stohl, A. & James, P. A Lagrangian analysis of the atmospheric branch of the global water cycle. Part I: Method description, validation, and demonstration for the August 2002 flooding in central Europe. J. Hydrometeorol. 5 , 656–678 (2004).
Petvirojchai, P. & SaraPa, S. Current technology for alerting and warning tropical cyclones in Thailand. Trop. Cyclone Res. Rev. 7 , 193–200 (2018).
Francis, J. A. & Vavrus, S. J. Evidence linking Arctic amplification to extreme weather in mid-latitudes. Geophys. Res. Lett . 39 , https://doi.org/10.1029/2012gl051000 (2012).
Hong, C.-C., Hsu, H.-H., Lin, N.-H. & Chiu, H. Roles of European blocking and tropical−extratropical interaction in the 2010 Pakistan flooding. Geophys. Res. Lett . 38 , https://doi.org/10.1029/2011gl047583 (2011).
Grams, C. M., Binder, H., Pfahl, S., Piaget, N. & Wernli, H. Atmospheric processes triggering the central European floods in June 2013. Nat. Hazards Earth Syst. Sci. 14 , 1691–1702 (2014).
Petrow, T., Merz, B., Lindenschmidt, K. E. & Thieken, A. H. Aspects of seasonality and flood generating circulation patterns in a mountainous catchment in south-eastern Germany. Hydrol. Earth Syst. Sci. 11 , 1455–1468 (2007).
Payne, A. E. et al. Responses and impacts of atmospheric rivers to climate change. Nat. Rev. Earth Environ. 1 , 143–157 (2020).
Ralph, F. M., Dettinger, M. D., Cairns, M. M., Galarneau, T. J. & Eylander, J. Defining “atmospheric river”: how the glossary of meteorology helped resolve a debate. Bull. Am. Meteorol. Soc. 99 , 837–839 (2018).
Dacre, H. F., Clark, P. A., Martinez-Alvarado, O., Stringer, M. A. & Lavers, D. A. How do atmospheric rivers form? Bull. Am. Meteorol. Soc. 96 , 1243–1255 (2015).
Young, A. M., Skelly, K. T. & Cordeira, J. M. High-impact hydrologic events and atmospheric rivers in California: an investigation using the NCEI Storm Events Database. Geophys. Res. Lett. 44 , 3393–3401 (2017).
Barth, N. A., Villarini, G., Nayak, M. A. & White, K. Mixed populations and annual flood frequency estimates in the western United States: the role of atmospheric rivers. Water Resour. Res. 53 , 257–269 (2017).
Lavers, D. A., Villarini, G., Allan, R. P., Wood, E. F. & Wade, A. J. The detection of atmospheric rivers in atmospheric reanalyses and their links to British winter floods and the large-scale climatic circulation. J. Geophys. Res. Atmos . 117 , https://doi.org/10.1029/2012JD018027 (2012).
Eiras-Barca, J. et al. The concurrence of atmospheric rivers and explosive cyclogenesis in the North Atlantic and North Pacific basins. Earth Syst. Dynam. 9 , 91–102 (2018).
Lu, M., Lall, U., Schwartz, A. & Kwon, H. Precipitation predictability associated with tropical moisture exports and circulation patterns for a major flood in France in 1995. Water Resour. Res. 49 , 6381–6392 (2013).
Dhana Lakshmi, D. & Satyanarayana, A. N. V. Influence of atmospheric rivers in the occurrence of devastating flood associated with extreme precipitation events over Chennai using different reanalysis data sets. Atmos. Res. 215 , 12–36 (2019).
Kingston, D. G., Lavers, D. A. & Hannah, D. M. Floods in the southern Alps of New Zealand: the importance of atmospheric rivers. Hydrol. Process. 30 , 5063–5070 (2016).
Porter, K. et al. Overview of the ARkStorm scenario. In U.S. Geological Survey Open-File Report 2010−1312 183 (USGS, 2011).
Porter, K., Cox, D., Dettinger, M. & Ralph, F. M. Special issue on the ARkStorm scenario: California’s other big one. Natural Haz. Rev . 17 , https://doi.org/10.1061/(ASCE)NH.1527-6996.0000234 (2016).
Kundzewicz, Z. W., Szwed, M. & Pińskwar, I. Climate variability and floods — a global review. Water 11 , 1399 (2019).
Waylen, P. R. & Caviedes, C. N. El Nino and annual floods on the north Peruvian littoral. J. Hydrol. 89 , 141–156 (1986).
Cai, W. et al. Climate impacts of the El Niño–Southern Oscillation on South America. Nat. Rev. Earth Environ. 1 , 215–231 (2020).
Kiem, A. S., Franks, S. W. & Kuczera, G. Multi-decadal variability of flood risk. Geophys. Res. Lett . 30 , https://doi.org/10.1029/2002GL015992 (2003).
Ward, P. J., Kummu, M. & Lall, U. Flood frequencies and durations and their response to El Niño Southern Oscillation: global analysis. J. Hydrol. 539 , 358–378 (2016).
Steirou, E., Gerlitz, L., Apel, H., Sun, X. & Merz, B. Climate influences on flood probabilities across Europe. Hydrol. Earth Syst. Sci. 23 , 1305–1322 (2019).
Delgado, J. M., Merz, B. & Apel, H. A climate-flood link for the lower Mekong River. Hydrol. Earth Syst. Sci. 16 , 1533–1541 (2012).
Messmer, M., Gómez-Navarro, J. J. & Raible, C. C. Climatology of Vb cyclones, physical mechanisms and their impact on extreme precipitation over Central Europe. Earth Syst. Dynam. 6 , 541–553 (2015).
Swierczynski, T. et al. Mid- to late Holocene flood frequency changes in the northeastern Alps as recorded in varved sediments of Lake Mondsee (Upper Austria). Quat. Sci. Rev. 80 , 78–90 (2013).
Hall, J. et al. Understanding flood regime changes in Europe: a state-of-the-art assessment. Hydrol. Earth Syst. Sci. 18 , 2735–2772 (2014).
Merz, B., Nguyen, V. D. & Vorogushyn, S. Temporal clustering of floods in Germany: do flood-rich and flood-poor periods exist? J. Hydrol. 541 , 824–838 (2016).
Hodgkins, G. A. et al. Climate-driven variability in the occurrence of major floods across North America and Europe. J. Hydrol. 552 , 704–717 (2017).
Lun, D., Fischer, S., Viglione, A. & Blöschl, G. Detecting flood-rich and flood-poor periods in annual peak discharges across Europe. Water Resour. Res. 56 , e2019WR026575 (2020).
Ward, P. J. et al. Strong influence of El Niño Southern Oscillation on flood risk around the world. Proc. Natl Acad. Sci. USA 111 , 15659–15664 (2014).
Nobre, G. G., Jongman, B., Aerts, J. & Ward, P. J. The role of climate variability in extreme floods in Europe. Environ. Res. Lett. 12 , 084012 (2017).
Zanardo, S., Nicotina, L., Hilberts, A. G. J. & Jewson, S. P. Modulation of economic losses from European floods by the North Atlantic Oscillation. Geophys. Res. Lett. 46 , 2563–2572 (2019).
Kwon, H.-H., Brown, C. & Lall, U. Climate informed flood frequency analysis and prediction in Montana using hierarchical Bayesian modeling. Geophys. Res. Lett. 35 , L05404 (2008).
Lima, C. H. R., Lall, U., Troy, T. J. & Devineni, N. A climate informed model for nonstationary flood risk prediction: application to Negro River at Manaus, Amazonia. J. Hydrol. 522 , 594–602 (2015).
Schröter, K., Kunz, M., Elmer, F., Mühr, B. & Merz, B. What made the June 2013 flood in Germany an exceptional event? A hydro-meteorological evaluation. Hydrol. Earth Syst. Sci. 19 , 309–327 (2015).
Merz, R. & Blöschl, G. Process controls on the statistical flood moments — a data based analysis. Hydrol. Process. 23 , 675–696 (2009).
Norbiato, D., Borga, M., Merz, R., Blöschl, G. & Carton, A. Controls on event runoff coefficients in the eastern Italian Alps. J. Hydrol. 375 , 312–325 (2009).
Bennett, B., Leonard, M., Deng, Y. & Westra, S. An empirical investigation into the effect of antecedent precipitation on flood volume. J. Hydrol. 567 , 435–445 (2018).
Tromp-van Meerveld, H. J. & McDonnell, J. J. Threshold relations in subsurface stormflow: 2. The fill and spill hypothesis. Water Resour. Res . 42 , https://doi.org/10.1029/2004wr003800 (2006).
Rogger, M. et al. Step changes in the flood frequency curve: process controls. Water Resour. Res . 48 , https://doi.org/10.1029/2011WR011187 (2012).
Rogger, M., Viglione, A., Derx, J. & Blöschl, G. Quantifying effects of catchments storage thresholds on step changes in the flood frequency curve. Water Resour. Res. 49 , 6946–6958 (2013).
Blöschl, G., Merz, R. & Reszler, C. Floods in Austria. In Extreme Hydrological Events: New Concepts for Security. NATO Science Series (eds Vasiliev, O., van Gelder, P., Plate, E. & Bolgov, M.) Vol. 78 (Springer, 2006).
Sivapalan, M., Blöschl, G., Merz, R. & Gutknecht, D. Linking flood frequency to long-term water balance: Incorporating effects of seasonality. Water Resour. Res . 41 , https://doi.org/10.1029/2004wr003439 (2005).
Blöschl, G., Nester, T., Komma, J., Parajka, J. & Perdigão, R. A. P. The June 2013 flood in the upper Danube basin, and comparisons with the 2002, 1954 and 1899 floods. Hydrol. Earth Syst. Sci. 17 , 5197–5212 (2013).
Guse, B. et al. The role of flood wave superposition in the severity of large floods. Hydrol. Earth Syst. Sci. 24 , 1633–1648 (2020).
Mirza, M. M. Q. Global warming and changes in the probability of occurrence of floods in Bangladesh and implications. Glob. Environ. Change 12 , 127–138 (2002).
O’Connell, E., Ewen, J., O’Donnell, G. & Quinn, P. Is there a link between agricultural land-use management and flooding? Hydrol. Earth Syst. Sci. 11 , 96–107 (2007).
Rogger, M. et al. Land use change impacts on floods at the catchment scale: challenges and opportunities for future research. Water Resour. Res. 53 , 5209–5219 (2017).
Bronstert, A. et al. Multi-scale modelling of land-use change and river training effects on floods in the Rhine basin. River Res. Appl. 23 , 1102–1125 (2007).
Te Linde, A. H., Aerts, J. C. J. H. & Kwadijk, J. C. J. Effectiveness of flood management measures on peak discharges in the Rhine basin under climate change. J. Flood Risk Manag. 3 , 248–269 (2010).
Hooijer, A., Klijn, F., Pedroli, G. B. M. & Van Os, A. G. Towards sustainable flood risk management in the Rhine and Meuse riverbasins: synopsis of the findings of IRMA-SPONGE. River Res.Applic. 20 , 343–357 (2004).
Salazar, S. et al. A comparative analysis of the effectiveness of flood management measures based on the concept of “retaining water in the landscape” in different European hydro-climatic regions. Natural Haz. Earth Syst. Sci. 12 , 3287–3306 (2012).
Pattison, I. & Lane, S. N. The link between land-use management and fluvial flood risk: a chaotic conception? Prog. Phys. Geogr. Earth Environ. 36 , 72–92 (2012).
Yang, L. et al. River networks system changes and its impact on storage and flood control capacity under rapid urbanization. Hydrol. Process. 30 , 2401–2412 (2016).
Best, J. Anthropogenic stresses on the world’s big rivers. Nat. Geosci. 12 , 7–21 (2019).
Van Khanh Triet, N. et al. Has dyke development in the Vietnamese Mekong Delta shifted flood hazard downstream? Hydrol. Earth Syst. Sci. 21 , 3991–4010 (2017).
Remo, J. W. F., Pinter, N. & Heine, R. The use of retro- and scenario-modeling to assess effects of 100+ years river of engineering and land-cover change on Middle and Lower Mississippi River flood stages. J. Hydrol. 376 , 403–416 (2009).
Apel, H., Merz, B. & Thieken, A. H. Influence of dike breaches on flood frequency estimation. Comput. Geosci. 35 , 907–923 (2009).
de Bruijn, K. M., Diermanse, F. L. M. & Beckers, J. V. L. An advanced method for flood risk analysis in river deltas, applied to societal flood fatality risk in the Netherlands. Natural Haz. Earth Syst. Sci. 14 , 2767–2781 (2014).
Graf, W. L. Downstream hydrologic and geomorphic effects of large dams on American rivers. Geomorphology 79 , 336–360 (2006).
Ayalew, T. B., Krajewski, W. F., Mantilla, R., Wright, D. B. & Small, S. J. Effect of spatially distributed small dams on flood frequency: insights from the Soap Creek watershed. J. Hydrol. Eng. 22 , 04017011 (2017).
Mei, X., Van Gelder, P. H. A. J. M., Dai, Z. & Tang, Z. Impact of dams on flood occurrence of selected rivers in the United States. Front. Earth Sci. 11 , 268–282 (2017).
Wang, W. et al. Nonlinear filtering effects of reservoirs on flood frequency curves at the regional scale. Water Resour. Res. 53 , 8277–8292 (2017).
Volpi, E., Di Lazzaro, M., Bertola, M., Viglione, A. & Fiori, A. Reservoir effects on flood peak discharge at the catchment scale. Water Resour. Res. 54 , 9623–9636 (2018).
Delle Rose, M. Decision-making errors and socio-political disputes over the Vajont dam disaster. Disaster Adv. 5 , 144–152 (2012).
Zscheischler, J. et al. A typology of compound weather and climate events. Nat. Rev. Earth Environ. 1 , 333–347 (2020).
Wahl, T., Jain, S., Bender, J., Meyers, S. D. & Luther, M. E. Increasing risk of compound flooding from storm surge and rainfall for major US cities. Nat. Clim. Change 5 , 093–1097 (2015).
Ganguli, P. & Merz, B. Extreme coastal water levels exacerbate fluvial flood hazards in northwestern Europe. Sci. Rep. 9 , 1–14 (2019).
Couasnon, A. et al. Measuring compound flood potential from river discharge and storm surge extremes at the global scale. Natural Haz. Earth Syst. Sci. 20 , 489–504 (2020).
Syvitski, J. P. et al. Sinking deltas due to human activities. Nat. Geosci. 2 , 681 (2009).
Ganguli, P., Paprotny, D., Hasan, M., Güntner, A. & Merz, B. Projected changes in compound flood hazard from riverine and coastal floods in northwestern Europe. Earth’s Future 8 , e2020EF001752 (2020).
Douben, K.-J. Characteristics of river floods and flooding: a global overview, 1985–2003. Irrig. Drain. 55 , S9–S21 (2006).
Kreibich, H. et al. Costing natural hazards. Nat. Clim. Change 4 , 303–306 (2014).
Allaire, M. Socio-economic impacts of flooding: a review of the empirical literature. Water Security 3 , 18–26 (2018).
Jonkman, S. N. Global perspectives on loss of human life caused by floods. Natural Haz. 34 , 151–175 (2005).
Hu, P., Zhang, Q., Shi, P. J., Chen, B. & Fang, J. Y. Flood-induced mortality across the globe: spatiotemporal pattern and influencing factors. Sci. Total. Environ. 643 , 171–182 (2018).
Jongman, B. et al. Declining vulnerability to river floods and the global benefits of adaptation. Proc. Natl Acad. Sci. USA 112 , E2271–E2280 (2015).
Jonkman, S. N. & Vrijling, J. K. Loss of life due to floods. J. Flood Risk Manag. 1 , 43–56 (2008).
Ashley, S. T. & Ashley, W. S. Flood fatalities in the United States. J. Appl. Meteorol. Climatol. 47 , 805–818 (2008).
Du, W., FitzGerald, G. J., Clark, M. & Hou, X.-Y. Health impacts of floods. Prehosp. Disaster Med. 25 , 265–272 (2012).
Elliott, J. R. Natural hazards and residential mobility: general patterns and racially unequal outcomes in the United States. Soc. Forces 93 , 1723–1747 (2014).
Moftakhari, H. R., AghaKouchak, A., Sanders, B. F., Allaire, M. & Matthew, R. A. What is nuisance flooding? Defining and monitoring an emerging challenge. Water Resour. Res. 54 , 4218–4227 (2018).
Merz, B., Elmer, F. & Thieken, A. H. Significance of “high probability/low damage” versus “low probability/high damage” flood events. Natural Haz. Earth Syst. Sci. 9 , 1033–1046 (2009).
World Meteorological Organization. Limpopo River basin: a proposal to improve the flood forecasting and early warning system. (WMO, 2012).
Koks, E. Moving flood risk modelling forwards. Nat. Clim. Change 8 , 561–562 (2018).
Haraguchi, M. & Lall, U. Flood risks and impacts: a case study of Thailand’s floods in 2011 and research questions for supply chain decision making. Int. J. Dis. Risk Reduct. 14 , 256–272 (2015).
World Economic Forum. Global risks 2012 and 2013. (WEF, 2013).
Helbing, D. Globally networked risks and how to respond. Nature 497 , 51–59 (2013).
United Nations Office for Disaster Risk Reduction. Global assessment report on disaster risk reduction. (UNDRR, 2019).
Weiping, W., Yang, S., Stanley, H. E. & Gao, J. Local floods induce large-scale abrupt failures of road networks. Nat. Commun. 10 , 2114 (2019).
Sieg, T. et al. Integrated assessment of short-term direct and indirect economic flood impacts including uncertainty quantification. PLoS ONE 14 , e0212932 (2019).
Thieken, A. H., Müller, M., Kreibich, H. & Merz, B. Flood damage and influencing factors: new insights from the August 2002 flood in Germany. Water Resour. Res. 41 , 1–16 (2005).
Merz, B., Kreibich, H., Schwarze, R. & Thieken, A. Review article “Assessment of economic flood damage”. Natural Haz. Earth Syst. Sci. 10 , 1697–1724 (2010).
Wing, O. E. J., Pinter, N., Bates, P. D. & Kousky, C. New insights into US flood vulnerability revealed from flood insurance big data. Nat. Commun. 11 , 1444 (2020).
Jonkman, S. N. & Kelman, I. An analysis of the causes and circumstances of flood disaster deaths. Disasters 29 , 75–97 (2005).
Bubeck, P., Botzen, W. J. W., Kreibich, H. & Aerts, J. C. J. H. Long-term development and effectiveness of private flood mitigation measures: an analysis for the German part of the river Rhine. Natural Haz. Earth Syst. Sci. 12 , 3507–3518 (2012b).
Haer, T., Botzen, W. J. W. & Aerts, J. C. J. H. Advancing disaster policies by integrating dynamic adaptive behaviour in risk assessments using an agent-based modelling approach. Environ. Res. Lett. 14 , 044022 (2019).
Kryspin-Watson, J., Dharmavaram, S., Stanton-Geddes, Z. & Chia, B. Urban Floods Community of Practice. Land use planning for urban flood risk management. (eds Himmelfarb, A. & Mora, J.) (World Bank, 2017).
United Nations International Strategy for Disaster Reduction . Sendai Framework for Disaster Risk Reduction 2015–2030 http://www.unisdr.org/files/43291_sendaiframeworkfordrren.pdf (UNISDR, 2015).
Tasantab, J. C. Beyond the plan: how land use control practices influence flood risk in Sekondi-Takoradi. JÀMBÁ J. Disaster Risk Stud. 11 , 1–9 (2019).
Brody, S. D., Zahran, S., Highfield, W. E., Bernhardt, S. P. & Vedlitz, A. Policy learning for flood mitigation: a longitudinal assessment of the community rating system in florida. Risk Anal. 29 , 912–929 (2009).
Miao, Q. Are we adapting to floods? Evidence from global flooding fatalities. Risk Anal. 39 , 1298–1313 (2019).
Kellens, W., Terpstra, T. & De Maeyer, P. Perception and communication of flood risks: a systematic review of empirical research. Risk Anal. 33 , 24–49 (2013).
Aerts, J. C. J. H. et al. Integrating human behaviour dynamics into flood disaster risk assessment. Nat. Clim. Change 8 , 193–199 (2018).
Kingdon, J. W. Agendas, Alternatives, and Public Policies 2nd edn (Longman, 1995).
Fleming, G. et al. Learning to live with rivers. Report of the Institution of Civil Engineers. https://web.archive.org/web/20110103063637/http://ice.org.uk/Information-resources/Document-Library/Learning-to-live-with-rivers (ICE, 2001).
EU directive 2007/60/EC of the European Parliament and of the Council of 23 October 2007 on the assessment and management of flood risks. The EU Floods Directive https://ec.europa.eu/environment/water/flood_risk (EU, 2007).
Kreibich, H. et al. Recent changes in flood preparedness of private households and businesses in Germany. Reg. Environ. Change 11 , 59–71 (2011).
Gallagher, J. Learning about an infrequent event: evidence from flood insurance take-up in the United States. Am. Econ. J. Appl. Econ. 6 , 206–233 (2014).
Atreya, A., Ferreira, S. & Kriesel, W. Forgetting the flood? An analysis of the flood risk discount over time. Land. Econ. 89 , 577–596 (2013).
Brouwer, R., Akter, S., Brander, L. & Haque, E. Socioeconomic vulnerability and adaptation to environmental risk: a case study of climate change and flooding in Bangladesh. Risk Anal. 27 , 313–326 (2007).
Karim, A. & Noy, I. Poverty and natural disasters: a regression meta-analysis. Rev. Econ. Inst . 7 , https://doi.org/10.5202/rei.v7i2.222 (2016).
Kellenberg, D. K. & Mobarak, A. M. Does rising income increase or decrease damage risk from natural disasters? J. Urban. Econ. 63 , 788–802 (2008).
Fankhauser, S. & McDermott, T. K. J. Understanding the adaptation deficit: why are poor countries more vulnerable to climate events than rich countries? Glob. Environ. Change 27 , 9–18 (2014).
Schumacher, I. & Strobl, E. Economic development and losses due to natural disasters: the role of hazard exposure. Ecol. Econ. 72 , 97–105 (2011).
Muttarak, R. & Lutz, W. Is education a key to reducing vulnerability to natural disasters and hence unavoidable climate change? Ecol. Soc . 19 , 42 (2014).
Milly, P. C. D., Wetherald, R. T., Dunne, K. A. & Delworth, T. L. Increasing risk of great floods in a changing climate. Nature 415 , 514–517 (2002).
Slater, L. et al. Global changes in 20-year, 50-year, and 100-year river floods. Geophys. Res. Lett. 48 , e2020GL091824 (2021).
Blöschl, G. et al. Changing climate both increases and decreases European river floods. Nature 573 , 108–111 (2019).
Slater, L. J. & Villarini, G. Recent trends in U.S. flood risk. Geophys. Res. Lett. 43 , 12428–12436 (2016).
Hirsch, R. M. & Ryberg, K. R. Has the magnitude of floods across the USA changed with global CO 2 levels? Hydrolog. Sci. J. 57 , 1–9 (2012).
Mallakpour, I. & Villarini, G. The changing nature of flooding across the central United States. Nat. Clim. Change 5 , 250–254 (2015).
Archfield, S. A., Hirsch, R. M., Viglione, A. & Blöschl, G. Fragmented patterns of flood change across the United States. Geophys. Res. Lett. 43 , 10232–10239 (2016).
Do, H. X., Westra, S. & Leonard, M. A global-scale investigation of trends in annual maximum streamflow. J. Hydrol. 552 , 28–43 (2017).
Gudmundsson, L., Leonard, M., Do, H. X., Westra, S. & Seneviratne, S. I. Observed trends in global indicators of mean and extreme streamflow. Geophys. Res. Lett. 46 , 756–766 (2019).
Bartiko, D., Oliveira, D. Y., Bonumá, N. B. & Chaffe, P. L. B. Spatial and seasonal patterns of flood change across Brazil. Hydrol. Sci. J. 64 , 1071–1079 (2019).
Ishak, E. & Rahman, A. Examination of changes in flood data in Australia. Water 11 , 1734 (2019).
Yang, L., Wang, L., Li, X. & Gao, J. On the flood peak distributions over China. Hydrol. Earth Syst. Sci. 23 , 5133–5149 (2019).
Merz, B., Vorogushyn, S., Uhlemann, S., Delgado, J. & Hundecha, Y. HESS Opinions: “More efforts and scientific rigour are needed to attribute trends in flood time series”. Hydrol. Earth Syst. Sci. 16 , 1379–1387 (2012).
Viglione, A. et al. Attribution of regional flood changes based on scaling fingerprints. Water Resour. Res. 52 , 5322–5340 (2016).
Kemter, M., Merz, B., Marwan, N., Vorogushyn, S. & Blöschl, G. Joint trends in flood magnitudes and spatial extents across Europe. Geophys. Res. Lett. 47 , e2020GL087464 (2020).
Intergovernmental Panel on Climate Change (IPCC). Managing the risks of extreme events and disasters to advance climate change adaptation. In A Special Report of Working Groups I and II of the Intergovernmental Panel on Climate Change (eds Field, C. B. et al.) 582 (Cambridge Univ. Press, 2012).
Screen, J. A. & Simmonds, I. Amplified mid-latitude planetary waves favour particular regional weather extremes. Nat. Clim. Change 4 , 704–709 (2014).
Petoukhov, V., Rahmstorf, S., Petri, S. & Schellnhuber, H. J. Quasiresonant amplification of planetary waves and recent Northern Hemisphere weather extremes. Proc. Natl Acad. Sci. USA 110 , 5336–5341 (2013).
Coumou, D., Di Capua, G., Vavrus, S., Wang, L. & Wang, S. The influence of Arctic amplification on mid-latitude summer circulation. Nat. Commun. 9 , 2959 (2018).
Stadtherr, L., Coumou, D., Petoukhov, V., Petri, S. & Rahmstorf, S. Record Balkan floods of 2014 linked to planetary wave resonance. Sci. Adv. 2 , e1501428 (2016).
Delgado, J. M., Apel, H. & Merz, B. Flood trends and variability in the Mekong river. Hydrol. Earth Syst. Sci. 14 , 407–418 (2010).
Bertola, M., Viglione, A., Lun, D., Hall, J. & Blöschl, G. Flood trends in Europe: are changes in small and big floods different? Hydrol. Earth Syst. Sci. 24 , 1805–1822 (2020).
Hosking, J. R. M. & Wallis, J. R. Regional Frequency Analysis: an Approach Based on L-Moments (Cambridge Univ. Press, 1997).
Dartmouth Flood Observatory. Space-based Measurement, Mapping, and Modeling of Surface Water For Research, Humanitarian, and Water Resources Applications http://floodobservatory.colorado.edu (DFO, 2020).
EmDAT. The CRED/OFDA International Disaster Database https://www.emdat.be/ (Université Catholique de Louvain, 2020).
DesInventar Sendai. Migrating DesInventar to Sendai Framework format. (United Nations Office for Disaster Risk Reduction, 2020).
SHELDUS. The spatial hazard events and losses database for the United States. Version 19. Center for Emergency Management and Homeland Security https://cemhs.asu.edu/sheldus (Arizona State University, 2020).
Kron, W., Steuer, M., Löw, P., & Wirtz, A. How to deal properly with a natural catastrophe database – analysis of flood losses. Nat. Hazards Earth Syst. Sci. , 12 , 535–550 https://doi.org/10.5194/nhess-12-535-2012 (2012).
Bouwer, L. M. & Jonkman, S. N. Global mortality from storm surges is decreasing. Environ. Res. Lett. 13 , 014008 (2018).
Di Baldassarre, G. et al. Flood fatalities in Africa: from diagnosis to mitigation. Geophys. Res. Lett . 37 , L22402 https://doi.org/10.1029/2010gl045467 (2010).
Paprotny, D., Sebastian, A., Morales-Nápoles, O. & Jonkman, S. N. Trends in flood losses in Europe over the past 150 years. Nat. Commun. 9 , 1985 (2018).
Bouwer, L. M. Have disaster losses increased due to anthropogenic climate change? Bull. Am. Meteorol. Soc. 92 , 39–46 (2011).
Visser, H., Petersen, A. C. & Ligtvoet, W. On the relation between weather-related disaster impacts, vulnerability and climate change. Clim. Change 125 , 461–477 (2014).
Kriegler, E. et al. The need for and use of socio-economic scenarios for climate change analysis: a new approach based on shared socio-economic pathways. Glob. Environ. Change 22 , 807–822 (2012).
Haer, T., Husby, T. G., Botzen, W. J. W. & Aerts, J. C. J. H. The safe development paradox: an agent-based model for flood risk under climate change in the European Union. Glob. Environ. Change 60 , 102009 (2020).
Ward, P. J. et al. A global framework for future costs and benefits of river-flood protection in urban areas. Nat. Clim. Change 7 , 642 (2017).
Kinoshita, Y., Tanoue, M., Watanabe, S. & Hirabayashi, Y. Quantifying the effect of autonomous adaptation to global river flood projections: application to future flood risk assessments. Environ. Res. Lett. 13 , 014006 (2018).
Willner, S. N., Levermann, A., Zhao, F. & Frieler, K. Adaptation required to preserve future high-end river flood risk at present levels. Sci. Adv . 4 , eaao1914 (2018).
Hirabayashi, Y. et al. Global flood risk under climate change. Nat. Clim. Change 3 , 816−821 (2013).
Winsemius, H. C. et al. Global drivers of future river flood risk. Nat. Clim. Change 6 , 381–385 (2016).
Alfieri, L. et al. Global projections of river flood risk in a warmer world. Earth’s Future 5 , 171–182 (2017).
Jiang, T. et al. Each 0.5 °C of warming increases annual flood losses in China by more than US$60 billion. Bull. Am. Meteorol. Soc. 101 , E1464–E1474 (2020).
Dankers, R. et al. First look at changes in flood hazard in the inter-sectoral impact model intercomparison project ensemble. Proc. Natl Acad. Sci. USA 111 , 3257–3261 (2014).
Arnell, N. W. & Gosling, S. N. The impacts of climate change on river flood risk at the global scale. Clim. Change 134 , 387–401 (2016).
Do, H. X. et al. Historical and future changes in global flood magnitude — evidence from a model–observation investigation. Hydrol. Earth Syst. Sci. 24 , 1543–1564 (2020).
Alfieri, L., Dottori, F., Betts, R., Salamon, P. & Feyen, L. Multi-model projections of river flood risk in Europe under global warming. Climate 6 , 6 (2018).
Shepherd, T. G. et al. Storylines: an alternative approach to representing uncertainty in physical aspects of climate change. Clim. Change 151 , 555–571 (2018).
Pfahl, S., O’Gorman, P. A. & Fischer, E. M. Understanding the regional pattern of projected future changes in extreme precipitation. Nat. Clim. Change 7 , 423–427 (2017).
Knutti, R., Masson, D. & Gettelman, A. Climate model genealogy: generation CMIP5 and how we got there. Geophys. Res. Lett. 40 , 1194–1199 (2013).
Scussolini, P. et al. FLOPROS: an evolving global database of flood protection standards. Natural Haz. Earth Syst. Sci. 16 , 1049–1061 (2016).
Jonkman, S. N. Advanced flood risk analysis required. Nat. Clim. Change 3 , 1004–1004 (2013).
Huizinga, J., de Moel, H. & Szewczyk, W. Global flood depth-damage functions. Methodology and the database with guidelines. EUR 28552 EN (European Union, 2017).
Sairam, N., Schröter, K., Lüdtke, S., Merz, B. & Kreibich, H. Quantifying flood vulnerability reduction via private precaution. Earth’s Future 7 , 235–249 (2019).
Lumbroso, D. Flood risk management in Africa. J. Flood Risk Manag. 13 , e12612 (2020).
March, J. G., Sproull, L. S. & Tamuz, M. Learning from samples of one or fewer. Org. Sci. 2 , 1–13 (1991).
Burton, I. Forensic disaster investigations in depth: a new case study model. Environ. Sci. Policy Sustain. Dev. 52 , 36–41 (2010).
Ciullo, A., Viglione, A., Castellarin, A., Crisci, M. & Di Baldassarre, G. Socio-hydrological modelling of flood-risk dynamics: comparing the resilience of green and technological systems. Hydrolog. Sci. J. 62 , 880–891 (2017).
Berner, C. L., Staid, A., Flage, R. & Guikema, S. D. The use of simulation to reduce the domain of “black swans” with application to hurricane impacts to power systems. Risk Anal. 37 , 1879–1897 (2017).
Wardekker, J. A., de Jong, A., Knoop, J. M. & van der Sluijs, J. P. Operationalising a resilience approach to adapting an urban delta to uncertain climate changes. Technol. Forecast. Soc. Change 77 , 987–998 (2010).
Merz, B., Vorogushyn, S., Lall, U., Viglione, A. & Blöschl, G. Charting unknown waters — on the role of surprise in flood risk assessment and management. Water Resour. Res. 51 , 6399–6416 (2015).
Parker, W. S. & Risbey, J. S. False precision, surprise and improved uncertainty assessment. Phil. Trans. R. Soc. A 373 , 20140453 (2015).
Jain, S. & Lall, U. Floods in a changing climate: does the past represent the future? Water Resour. Res. 37 , 3193–3205 (2001).
Leonard, M. et al. A compound event framework for understanding extreme impacts. Wiley Interdisc. Rev. Clim. Change 5 , 113–128 (2014).
Kahneman, D. Thinking, Fast and Slow 499 (Farrar, Straus and Giroux, 2011).
Woo, G. Downward counterfactual search for extreme events. Front. Earth Sci . https://doi.org/10.3389/feart.2019.00340 (2019).
Brysse, K., Oreskes, N., O’Reilly, J. & Oppenheimer, M. Climate change prediction: erring on the side of least drama? Glob. Environ. Change 23 , 327–337 (2013).
Croskerry, P., Singhal, G. & Mamede, S. Cognitive debiasing. 1: Origins of bias and theory of debiasing. BMJ Qual. Saf. 22 , ii58–ii64 (2013).
Taleb, N. N. The Black Swan: The Impact of the Highly Improbable 444 (Random House, 2007).
Ben-Haim, Y. Why risk analysis is difficult, and some thoughts on how to proceed. Risk Anal. 32 , 1638–1646 (2012).
IRGC. Introduction to the IRGC Risk Governance Framework, revised version 2017. (EPFL International Risk Governance Center, 2017).
Kwakkel, J. H., Walker, W. E. & Haasnoot, M. Coping with the wickedness of public policy problems: approaches for decision making under deep uncertainty. J. Water Resour. Plan. Manag. 142 , 01816001 (2016).
Simpson, M. et al. Decision analysis for management of natural hazards. Annu. Rev. Environ. Resour. 41 , 489–516 (2016).
Lempert, R. et al. Ensuring Robust Flood Risk Management in Ho Chi Minh City. World Bank Policy Research Working Paper No. 6465 https://papers.ssrn.com/sol3/papers.cfm?abstract_id=2271955 (SSRN, 2013).
Kalra, N. et al. Agreeing on robust decisions: new processes for decision making under deep uncertainty. Policy Research Working Paper No. 6906. 35 (World Bank, 2014).
Sayers, P. et al. Flood Risk Management: A Strategic Approach (UNESCO, 2013).
Haasnoot, M., Kwakkel, J. H., Walker, W. E. & ter Maat, J. Dynamic adaptive policy pathways: a method for crafting robust decisions for a deeply uncertain world. Glob. Environ. Change 23 , 485–498 (2013).
Korteling, B., Dessai, S. & Kapelan, Z. Using information-gap decision theory for water resources planning under severe uncertainty. Water Resour. Manag. 27 , 1149–1172 (2013).
Brown, C., Ghile, Y., Laverty, M. & Li, K. Decision scaling: linking bottom-up vulnerability analysis with climate projections in the water sector. Water Resour. Res. 48 , W09537 (2012).
Restemeyer, B., van den Brink, M. & Woltjer, J. Resilience unpacked — framing of ‘uncertainty’ and ‘adaptability’ in long-term flood risk management strategies for London and Rotterdam. Eur. Plan. Stud. 26 , 1559–1579 (2018).
Disse, M., Johnson, T. G., Leandro, J. & Hartmann, T. Exploring the relation between flood risk management and flood resilience. Water Secur. 9 , 100059 (2020).
Sen, P. K. Estimates of the regression coefficient based on Kendall’s tau. J. Am. Stat. Assoc. 63 , 1379–1389 (1968).
Kriegler, E., et al. Fossil-fueled development (SSP5): An energy and resource intensive scenario for the 21st century. Global Environmental Change 42 , 297–315 (2017).
Gall, M., Borden, K. A. & Cutter, S. L. When do losses count?: Six fallacies of natural hazards loss data. Bull. Am. Meteorol. Soc. 90 , 799–810 (2009).
Panwar, V. & Sen, S. Disaster damage records of EM-DAT and DesInventar: a systematic comparison. Econ. Dis. Clim. Change 4 , 295–317 (2020).
Adelekan, I. O. Flood risk management in the coastal city of Lagos, Nigeria. J. Flood Risk Manag. 9 , 255–264 (2016).
Download references
Acknowledgements
This work was supported by the DFG projects ‘SPATE’ (FOR 2416) and ‘NatRiskChange’ (GRK 2043/1), the FWF ‘SPATE’ project (I 3174), the ERC Advanced Grant ‘FloodChange’ project (number 291152), the Horizon 2020 ETN ‘System Risk’ project (number 676027) and the Helmholtz Climate Initiative. P.B. was supported by a Royal Society Wolfson Research Merit award. J.C.J.H.A. was supported by an ERC Advanced Grant COASTMOVE (number 884442) and a NWO-VICI grant (number 453-13-006).
Author information
Authors and affiliations.
Section Hydrology, Helmholtz Centre Potsdam, GFZ German Research Centre for Geosciences, Potsdam, Germany
Bruno Merz, Sergiy Vorogushyn, Matthias Kemter, Heidi Kreibich & Elena Macdonald
Institute for Environmental Sciences and Geography, University of Potsdam, Potsdam, Germany
Bruno Merz & Matthias Kemter
Institute of Hydraulic Engineering and Water Resources Management, Vienna University of Technology, Vienna, Austria
Günter Blöschl & Miriam Bertola
European Commission, Joint Research Centre, Ispra, Italy
Francesco Dottori
Institute for Environmental Studies (IVM), Amsterdam, The Netherlands
- Jeroen C. J. H. Aerts
Deltares, Delft, The Netherlands
School of Geographical Sciences, Bristol University, Bristol, UK
Potsdam Institute for Climate Impact Research (PIK), Potsdam, Germany
Matthias Kemter
Earth and Environmental Engineering, Colombia Water Center, Columbia University, New York, NY, USA
Upmanu Lall
You can also search for this author in PubMed Google Scholar
Contributions
B.M. suggested the original concept and coordinated the writing. G.B., S.V. and F.D. made major contributions to the writing. B.M., M.K., E.M. and S.V. generated the figures. All authors discussed the concepts and contributed to the writing.
Corresponding author
Correspondence to Bruno Merz .
Ethics declarations
Competing interests.
The authors declare no competing interests.
Additional information
Peer review information.
Nature Reviews Earth & Environment thanks Seth Westra and the other, anonymous, reviewer(s) for their contribution to the peer review of this work.
Publisher’s note
Springer Nature remains neutral with regard to jurisdictional claims in published maps and institutional affiliations.
Supplementary information
Supplementary information.
(AAL). A widespread indicator for risk, it is the estimated average loss per year considering the full range of scenarios from frequent events (zero or small loss) to extreme events (large loss or worst-case scenario).
Fall of rain onto existing snow, leading to flood runoff composed of snowmelt and rainfall.
Long, narrow and transient corridors of strong horizontal water vapour, transporting on average more than double the flow of the Amazon river and delivering moisture as heavy precipitation.
The fraction of the event water input (precipitation or snowmelt within the catchment) that is not retained in the catchment and that directly contributes to discharge during the event.
Consequences occurring in the inundated region during a flooding event.
Consequences occurring far away from the flooded region and/or after a flooding event.
Consequences of a flooding event that are difficult or impossible to monetarize, such as loss of life or loss of memorabilia.
The ratio of the number of people who lose their lives in a flood to the number of people affected by the flooding event.
The highest streamflow peak in each year.
The dates of the year when floods occur.
The distance over which flooding occurs simultaneously.
Level at which a flood causes extensive inundation, significant evacuations, or property transfer to higher ground.
Level at which a flood does not cause damage but requires mitigation action in preparation for more substantial flooding.
According to the DFO, either the total number of people left homeless after the incident, or the number of people evacuated during the flood.
Coupled Model Intercomparison Project Phase 5; for coordinated climate change experiments for the Fifth Assessment Report AR5 of the Intergovernmental Panel on Climate Change and beyond.
An indicator expressing the exceedance probability or rarity of an event. For instance, a 100-year flood discharge has a probability of 1/100 of being exceeded in a given year.
Relation between flood discharge and the associated return period.
Optimizing risk reduction measures based on the best available knowledge.
Rights and permissions
Reprints and permissions
About this article
Cite this article.
Merz, B., Blöschl, G., Vorogushyn, S. et al. Causes, impacts and patterns of disastrous river floods. Nat Rev Earth Environ 2 , 592–609 (2021). https://doi.org/10.1038/s43017-021-00195-3
Download citation
Accepted : 16 June 2021
Published : 10 August 2021
Issue Date : September 2021
DOI : https://doi.org/10.1038/s43017-021-00195-3
Share this article
Anyone you share the following link with will be able to read this content:
Sorry, a shareable link is not currently available for this article.
Provided by the Springer Nature SharedIt content-sharing initiative
This article is cited by
Flood teleconnections from levees undermine disaster resilience.
- Abolfazl Hojjat Ansari
- Alfonso Mejia
npj Natural Hazards (2024)
Higher emissions scenarios lead to more extreme flooding in the United States
- Hanbeen Kim
- Gabriele Villarini
Nature Communications (2024)
High-resolution impact-based early warning system for riverine flooding
- Husain Najafi
- Pallav Kumar Shrestha
- Luis Samaniego
Stochastic coastal flood risk modelling for the east coast of Africa
- Irene Benito
Historical trends and future implications of disasters in Honduras
- Adolfo Quesada-Román
- Lidia Torres-Bernhard
- Natalia Martínez-Rojas
Natural Hazards (2024)
Quick links
- Explore articles by subject
- Guide to authors
- Editorial policies
Sign up for the Nature Briefing newsletter — what matters in science, free to your inbox daily.

- Comments This field is for validation purposes and should be left unchanged.
- Climate Change
- Policy & Economics
- Biodiversity
- Conservation
Get focused newsletters especially designed to be concise and easy to digest
- ESSENTIAL BRIEFING 3 times weekly
- TOP STORY ROUNDUP Once a week
- MONTHLY OVERVIEW Once a month
- Enter your email *
What Are the Main Causes and Effects of Floods Around the World?
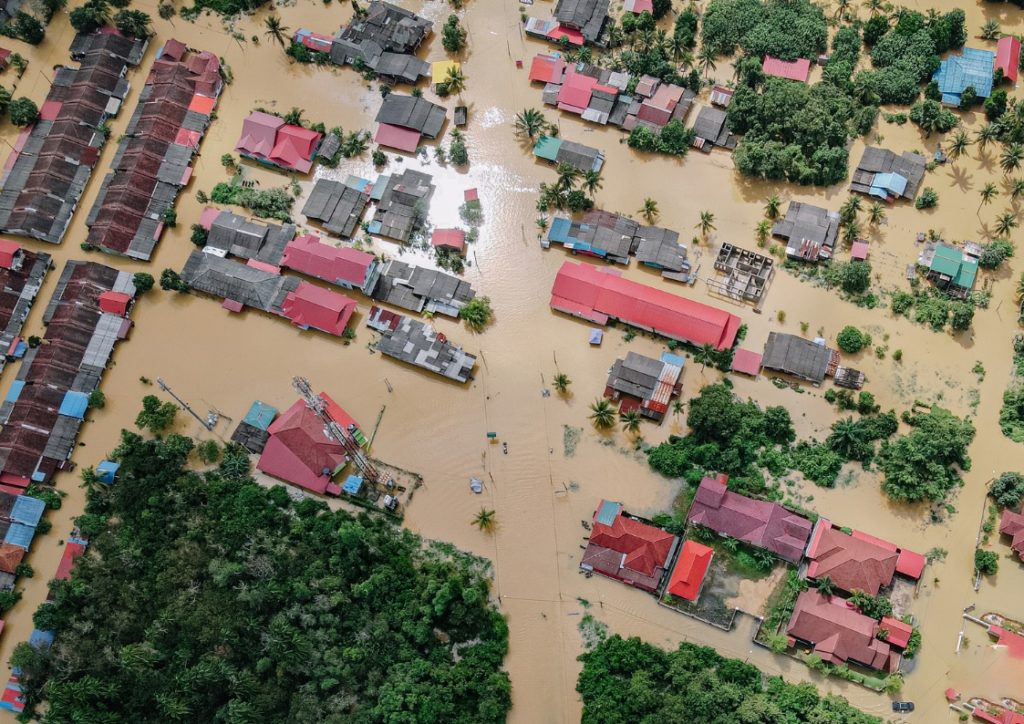
Floods are among the most devastating natural disasters that occur worldwide, affecting millions of people and causing significant damage to both human settlements and the environment. As our planet experiences the impacts of climate change and human activities continue to alter landscapes, understanding the main causes and effects of floods becomes increasingly crucial. In this article, we delve into the factors that contribute to flooding and explore the wide-ranging consequences it has on our ecosystems and communities.
What Are the Different Types of Floods?
Before we examine the causes and effects of floods, it’s important to note that various types of floods can occur worldwide. There are two types of floods that are most common: flash floods and river floods.
Flash floods, as the name suggests, are the rapid rise of water levels from excessive rainfall in low-lying areas. These weather events are incredibly dangerous and can often lead to fatalities due to their destructive power and incredible speed, often not giving people enough time to escape to higher grounds or adopt protective measures. Flash floods tend to be more common in areas with a dry climate and rocky terrain due to lack of soil or vegetation, which acts as a defence or barrier against torrential rains flowing overland.
River flooding on the other hand, occurs when a river overspills its banks and river waters can no longer be contained within its channel. These events are more common in areas with a wetter climate and have longer rainstorm seasons, as well as areas close to melting snow and ice.
What Are the Main Causes of Floods?
Floods can occur due to several different, and often simultaneous, factors. However, one of the biggest causes of floods, especially in cases of flash floods, is excessive and heavy rainfall. When rainfall in low-lying areas and urban environments fall faster than the ground can absorb, water height rapidly rise, resulting in floods. Extreme rainfall in river courses contribute to flooding as well, as water travels down and overflows riverbanks onto surrounding land.
Sea overflow can also cause floods, in an event also known as a storm surge. This occurs during tropical storms, cyclones and hurricanes, where these types of weather events cause sea water to overflow onto the land in coastal regions. Sea water levels have been recorded to rise as high as 20 feet during storm surges.
Rapid melting of snow and ice similarly causes a surge of sea water, while blocks of melting ice could block the flow of a river, creating a phenomenon known as ice jams.
Dam failures and breakage can also send a powerful and destructive surge of water downstream. One of the most devastating dam failures in history took place in 1889 in Johnstown, Pennsylvania . Several days of extraordinarily heavy rain placed severe pressure on the local dam, causing it to fail and releasing 20 million tons of water to the town, and more than 2,200 people died within minutes.
What Are the Biggest Effects of Floods?
More than 2 billion people worldwide were affected by floods between 1998-2017. And that number continues to climb as occurrences of major flood events become more frequent and severe.
Flooding, especially flash floods, can devastate entire cities and urban environments. Throughout history, many have lost their lives as a result of rapid floods, or events caused by severe flooding such as landslides and collapsed infrastructure.
One of the biggest consequences and effects of floods is that people lose their home and property, and essential buildings and infrastructures such as hospitals and elderly homes are left incapacitated. Loss of power and mobile communication is a common occurrence during floods, which can impact livelihoods and access to safety.
Floods can have huge economic repercussions to a region, as extreme weather events impact key industries and sectors, notably agriculture, fishing, food crops, health, labour and tourism. Studies have found that frequent flooding could shave 11% off a region’s GDP by the end of the century. Countries often take years to recover economically following the loss of resources.
People who live in regions close to rivers, in wetter climates and prone to monsoon seasons are significantly more vulnerable to floods. Many south and southeast Asian countries such as Bangladesh – a third of which was under water at one point in 2020 – and India have been particularly hit hard by flood events in recent years due to their low-lying lands and dense populations.
Consequently, there’s been high rates of mass migration and population displacement over the past few decades, causing overcrowding in urban cities and expanding the urban poor. This leads to potential long-term social inequalities and unrest.
You might also like: 86 Million People Have Been Moving Into Global Flood Zones in the Last Two Decades – Study
Climate Change is Exacerbating the Effects of Floods
Flooding has been made more likely by climate change , according to a 2021 study by climate experts. In the case of Western Europe, downpours in the region, which caused the flash floods that killed nearly 200 people, are now 3-19% heavier due to human-caused warming.
For countries that are already prone to rainy seasons, particularly in Asia, climate models predict climate change will lead to more intense flooding and prolong existing monsoon seasons . A typical monsoon season in Asia lasts from June to September. The effects of climate change could potentially result in an earlier arrival or departure of monsoons, disrupting agricultural and crop production, as well as increasing extreme precipitation over the region as more greenhouse gases are released into the atmosphere.
You might also like: Climate Change Made Libya Floods Up to 50 Times More Likely, Study Finds

What Can Be Done?
One of the most salient short-term solutions is for countries, especially those in high flood risk regions, to prioritise investments in flood adaptation strategies. This means building more resilient infrastructure that are designed to withstand floods and water damage.
China, for example, has been investing heavily in ‘sponge city’ concepts , the development of which could potentially help control and mitigate flooding, and recycle rainwater resources and re-instate degraded environments at the same time. Building seawalls can be an effective form of coastal defence and protection in dealing with storm surges and flooding events associated with tropical storms.
Managed retreat, which refers to a purposeful and coordinated movement of people and infrastructure away from high risk areas, is a solution that is worth considering. With more frequent and intense flooding events, forced migration and population displacement is already a growing problem, which as discussed earlier, brings with it a wealth of complex social and economic issues. A managed retreat, which can occur over time and allow systems that account for migrants and displaced people to be in place, can be less traumatic for people and potentially less expensive in the long run.
However ultimately, the most effective way to mitigate climate change and worsening flooding events is to reduce global greenhouse gas emissions. We need to dedicate ourselves to decarbonising transportation and investing a lot more and faster into renewable energy and technologies. While many high income countries such as the US have made net zero goals by 2050 and are pushing for more electric vehicles , a majority of the countries are doing far too little and at too slow a pace to tackle the exacerbating climate crisis.
This story is funded by readers like you
Our non-profit newsroom provides climate coverage free of charge and advertising. Your one-off or monthly donations play a crucial role in supporting our operations, expanding our reach, and maintaining our editorial independence.
About EO | Mission Statement | Impact & Reach | Write for us
About the Author

15 Biggest Environmental Problems of 2024
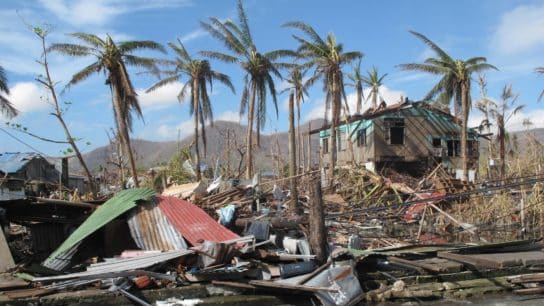
4 Biggest Environmental Issues in the Philippines in 2024
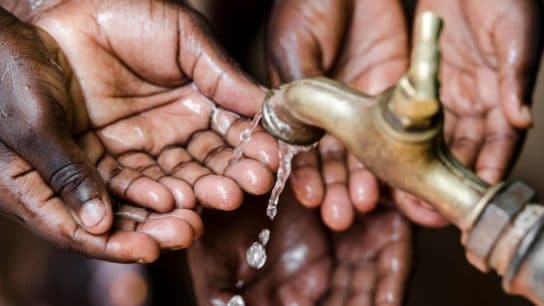
Water Shortage: Causes and Effects
Hand-picked stories weekly or monthly. We promise, no spam!
- Name This field is for validation purposes and should be left unchanged.
Boost this article By donating us $100, $50 or subscribe to Boosting $10/month – we can get this article and others in front of tens of thousands of specially targeted readers. This targeted Boosting – helps us to reach wider audiences – aiming to convince the unconvinced, to inform the uninformed, to enlighten the dogmatic.

- TOP CATEGORIES
- AS and A Level
- University Degree
- International Baccalaureate
- Uncategorised
- 5 Star Essays
- Study Tools
- Study Guides
- Meet the Team
Rivers: Case study of the Mississippi Floods, 1993
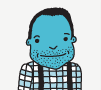
Use our dedicated case study to learn in-depth about the Mississippi Floods, April to October 1993.
- KEY CONCEPTS
- FLOOD MANAGEMENT
Introduction
The Mississippi river drains an area of nearly 3 million square kilometres and is the fifth largest river basin in the world. It provides a good case study as there are a range of human and physical causes, huge impacts of flooding and some good examples of effective action taken as a result. The river floodplain is up to 200km wide in the middle stages of the river’s course and as you would expect, there has been a lot of development of the area with much urbanisation taking place. The river has been managed for many years by hard and soft engineering techniques, channel straightening has been used extensively and has reduce the length of the river by 150 miles for example.
The flood was one of the worst in American history with dams bursting, levees being breached and the river remaining at a height above flood level for nearly 150 days. In total, nearly 78,000 square kilometres of land were flooded.
Background essays
Mississippi Flood 1993: Case Study
The Mississippi River, located in North America, begins in Lake Itasca, Minnesota and flows south...
Physical causes:
High rainfall towards the back end of 1992 meant that the soil in the basin was holding more water than usual and in many parts the ground was completely saturated . The soil’s capacity to hold water was therefore very low. There was more snow that usual in the winter from later 1992 to 1993, this built up stores of water that potentially would enter the river during the snowmelt season. From the late spring there were a large number of storms and the area suffered persistent rainfall . As much of the ground was saturated and the water table was high , water entered the river channels very quickly as surface run-off or overland flow. There were huge amounts of rainfall in 24 hour periods across the basin, up to 180mm was not uncommon. Many areas of the basin experienced up to 6 times the usual amount of precipitation. Heavy rainfall in the highest reaches of the snow covered areas of the basin accelerated snowmelt and this had a huge impact on the volume of water entering the channel.
Human Causes:
Shortening of the river’s channel by 150 miles over many years had a cumulative effect of pushing water downstream more quickly, especially during times of peak flow. Extensive dredging had taken place to keep the channel sediment free to improve navigation, the river is a significant part of the transport infrastructure of the USA. 75% of the wetland areas of the river basin had been urbanised impacting on the time taken for water to enter the river system. The wetlands ability to act as a store and release the water slowly into streams and rivers was virtually destroyed. Once flood warning were issued and areas were advised to be evacuated, many people thought that they were not at risk because there were so many protection measures in place. Once the river did flood then there was more damage and danger to people who had stayed in their homes and businesses.
Essays on the causes
'The 1993 Mississippi floods were caused by hard river engineering'. Discuss this statement.
The 1993 Mississippi Flood Report.
Socio-economic
The flood cost an estimated US $15 billion dollars in damages and over 800,000km2 were affected. 30,000 people had no clean water supply until it could be restored by engineers, 72,000 homes were flooded and close to 48,000 were evacuated from their homes. 45 people died as a direct result of the flood.
Flood defences were destroyed completely or damaged. Farmers lost an estimated US $2.5 billion of crops. Much of the flooded farmland was rendered useless for years after the event as it was so badly affected. Infrastructure was seriously affected with roads, bridges, railway and of course river traffic affected. This had a knock on effect to the economy as close to 10,000 were made jobless as a result of their place of work being destroyed.
Environmental
There was a huge loss of natural habitat and wetland areas which were important breeding grounds for bird and animal life having a long term effect on the ecosystem and biodiversity. The habitat for fauna that lives in the water improved due to increased areas of protection and shelter (much like a shipwreck in the sea provides a haven for marine life). Wildlife did have to contend with contaminated water though as chemicals from farms, stores and industry were dissolved into the floodwater. This also affected human drinking water supply.
After the initial flooding there was a lot of standing and stagnant water which attracted insects including mosquitoes, and rats were a problem in some areas.
Flood management since 1993
Since the flooding subsided the US government prioritised the management of the area affected and much engineering has taken place to try and reduce the risk of future flooding. The 6 very large dams built along the Mississippi’s main channel have been added to by building more on the tributaries. There are 9 dams on the Tennessee (a major tributary of the Mississippi) and a further ten on its own tributaries.
A series of 105 reservoirs have been linked to control the risk of flooding by controlling the flow of water through them.
Afforestation has been prominent in some areas to slow down the rate at which precipitation or snowmelt enters the channel system.
Although straightening and shortening of the river added to the problems of the 1993 flooding, more of this has been done and engineers have learned from previous experience. Levees have been reinforced with concrete rather than more natural and weaker methods (soil for example) and raised to more than the level of the 1993 flood waters. These levees provide protection for 1000’s of settlements along the Mississippi’s course.
Essays on the impact
Flooding on the Mississippi
River Management Case Study on The Mississippi.
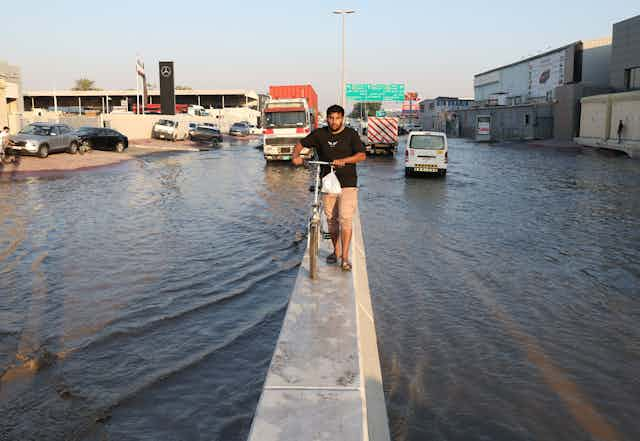
The weather experiment that really flooded Dubai
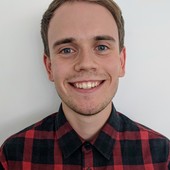
Environment + Energy Editor, UK edition
View all partners
A reckless experiment in Earth’s atmosphere caused a desert metropolis to flood.
That was the story last week when more than a year’s worth of rain fell in a day on the Arabian Peninsula, one of the world’s driest regions. Desert cities like Dubai in the United Arab Emirates (UAE) suffered floods that submerged motorways and airport runways. Across UAE and Oman, 21 people lost their lives.
The heavy rain of Tuesday April 16 was initially blamed on “cloud seeding”: a method of stimulating precipitation by injecting clouds with tiny particles that moisture can attach to – those droplets then merge and multiply. As the waters receded, however, a more disturbing explanation emerged.
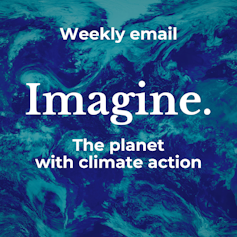
This roundup of The Conversation’s climate coverage comes from our weekly climate action newsletter . Every Wednesday, The Conversation’s environment editor writes Imagine, a short email that goes a little deeper into just one climate issue. Join the 30,000+ readers who’ve subscribed.
Richard Washington, a professor of climate science at the University of Oxford, has seen the inside of a storm. To confirm if cloud seeding really could breed record-breaking rain , he once boarded an aeroplane bound for a thundercloud over the South Africa-Mozambique border.
Read more: Don't blame Dubai's freak rain on cloud seeding – the storm was far too big to be human-made
“Our mission was to fly through the most active part of the storm, measure it, fly through again while dumping a bin load of dry ice, turn hard and fly through for a final measurement,” he says.
“Apart from the fun of flying through the core of a thunderstorm in a Learjet, I didn’t think much about the time I was lucky enough to be part of that project. Until I heard about the recent freak storm in Dubai.”
What caused the flood?
There are no two identical clouds with which to compare the outcome of seeding, Washington says, so it’s impossible to prove if this technique can change the outcome of a single storm. But by flying a lot of missions, half with cloud seeding and half without, and measuring rainfall between the two, meteorologists eventually showed that cloud seeding did modify rain rates in some storms.
That’s not what caused Dubai’s floods though.
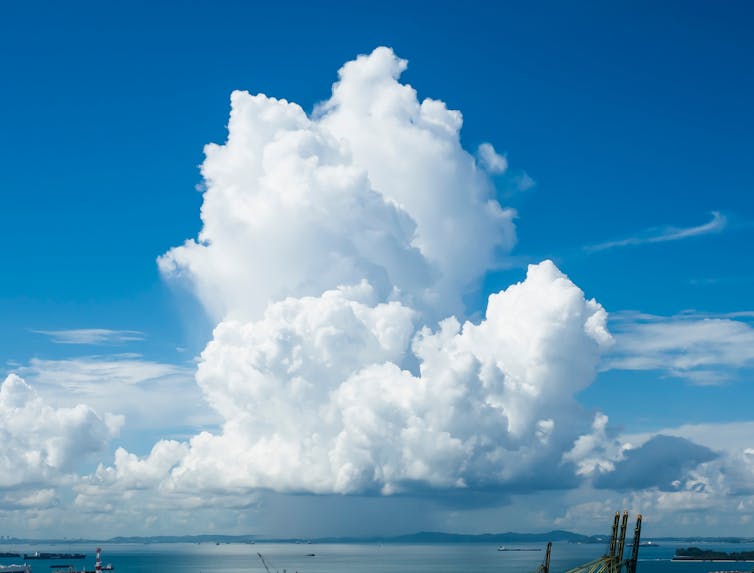
“It turns out the UAE has been running a cloud seeding project, UAE Research Program for Rain Enhancement Science, for several years. Their approach is to fire hygroscopic (water-attracting) salt flares from aircraft into warm cumuliform clouds,” Washington says.
“So could seeding have built a huge storm system the size of France? Let’s be clear, that would be like a breeze stopping an intercity train going at full tilt. And the seeding flights had not happened that day either. The kind of deep, large-scale clouds formed on April 16 are not the target of the experiment.”
For Washington, the more relevant atmospheric experiment is the one each of us is engaged in everyday.
“The interesting thing is that humans have a hard time coming to terms with the fact that 2,400 gigatonnes of carbon (our total emissions since pre-industrial times) might make a difference to the climate, but very readily get behind the idea of a few hygroscopic flares making 18 months worth of rain fall in a day.”
The experiment of our lives
A hotter atmosphere holds more moisture, which can fall as rain. Although last week’s deluge was unusual, the Arabian Peninsula does tend to receive more of its precipitation in heavy bursts than steady showers.
The latest assessment by the Intergovernmental Panel on Climate Change (IPCC) did not predict future rainfall trends for the region but did say global heating is expected to make such violent downpours more frequent and severe.
What is likely to kill more people as temperatures rise in this part of the world is not water, but heat. Tom Matthews (Loughborough University) and Colin Raymond (California Institute of Technology) are scientists who study the shifting climate and its effect on our bodies .
Read more: Global warming now pushing heat into territory humans cannot tolerate
Throughout human evolution, the wet-bulb temperature (how hot it is when you subtract the cooling effect of evaporating moisture, like sweat on your skin) has rarely, if ever, strayed beyond 35°C. At this threshold the air is so hot and humid that you cannot lower your temperature to a safe level by sweating. You overheat and, without urgent medical aid, die.
“The frequency of punishing wet-bulb temperatures has more than doubled worldwide since 1979, and in some of the hottest and most humid places on Earth, like the coastal United Arab Emirates, wet-bulb temperatures have already flickered past 35°C,” Matthews and Raymond say.
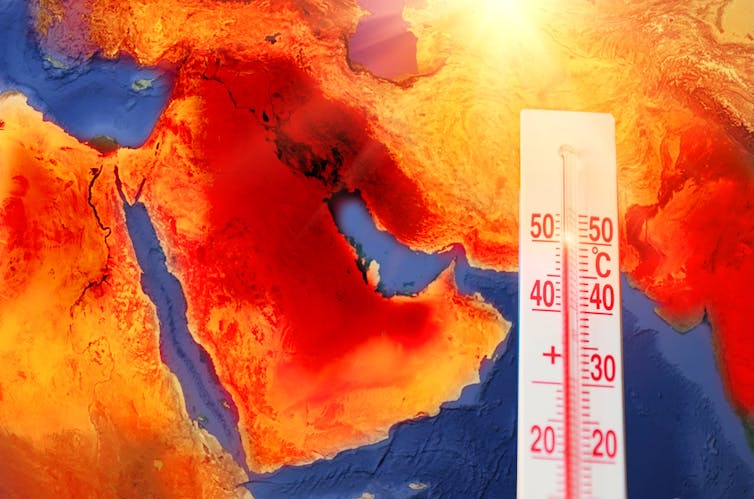
“The climate envelope is pushing into territory where our physiology cannot follow.”
Alarmed by how fast we are making the climate unlivable, some scientists have called for emergency measures. Peter Irvine, a lecturer in earth sciences at UCL, proposes dimming the sun by pumping microscopic particles into the upper atmosphere to reflect some of its rays.
Read more: Why dimming the Sun would be an effective tool in the fight against climate change
Trying to mimic the cooling effect of a volcanic eruption but on a permanent basis (until, presumably, greenhouse gas concentrations can be returned to safe levels) is another gamble with the atmosphere. These layers of gases that surround our planet have nurtured life by keeping temperatures stable and harmful radiation out.
Irvine acknowledges that keeping Earth artificially cool this way is risky, but argues the side effects – like altered wind and rainfall patterns, acid rain and delayed ozone layer recovery – “pale in comparison to the impacts of climate change”.
Catriona McKinnon, a professor of political theory at the University of Reading, has other concerns about attempting to manage solar radiation this way, including the question of who has the right to regulate the global thermostat.
Read more: Time is running out on climate change, but geoengineering has dangers of its own
As humanity contemplates another large-scale experiment in our atmosphere, there is another, even bigger one, waiting to be resolved. Its solution is simple: stop burning fossil fuels.
- Climate change
- Cloud seeding
- Imagine newsletter
Postdoctoral Research Associate

Project Manager – Contraceptive Development

Editorial Internship

Integrated Management of Invasive Pampas Grass for Enhanced Land Rehabilitation
Flooding Case studies
Cockermouth, UK – Rich Country (MEDC) Picture Causes: Rain A massive downpour of rain (31.4cm), over a 24-hour period triggered the floods that hit Cockermouth and Workington in Cumbria in November 2009
What caused all the rain? The long downpour was caused by a lengthy flow of warm, moist air that came down from the Azores in the mid-Atlantic. This kind of airflow is common in the UK during autumn and winter, and is known as a ‘warm conveyor’. The warmer the air is, the more moisture it can hold.
What else helped to cause the Cumbrian Floods? · The ground was already saturated, so the additional rain flowed as surface run-off straight into the rivers · The steep slopes of the Cumbrian Mountains helped the water to run very rapidly into the rivers · The rivers Derwent and Cocker were already swollen with previous rainfall · Cockermouth is at the confluence of the Derwent and Cocker (i.e. they meet there)
The effects of the flood · Over 1300 homes were flooded and contaminated with sewage · A number of people had to be evacuated, including 50 by helicopter, when the flooding cut off Cockermouth town centre · Many businesses were flooded causing long-term difficulties for the local economy · People were told that they were unlikely to be able to move back into flood-damaged homes for at least a year. The cost of putting right the damage was an average of £28,000 per house · Insurance companies estimated that the final cost of the flood could reach £100 million · Four bridges collapsed and 12 were closed because of flood damage. In Workington, all the bridges were destroyed or so badly damaged that they were declared unsafe – cutting the town in two. People faced a huge round trip to get from one side of the town to the other, using safe bridges · One man died– PC Bill Barker
Responses to the flood · The government provided £1 million to help with the clean-up and repairs and agreed to pay for road and bridge repairs in Cumbria · The Cumbria Flood Recovery Fund was set up to help victims of the flood. It reached £1 million after just 10 days · Network Rail opened a temporary railway station in Workington The ‘Visit Cumbria’ website provided lists of recovery services and trades, and people who could provide emergency accommodation
Management of future floods at Cockermouth £4.4 million pound management scheme New flood defence walls will halt the spread of the river Funding from Government and local contributors River dredged more regularly to deepen the channel New embankments raise the channel height to reduce the likelihood of extra floods New floodgates at the back of houses in Waterloo street
Pakistan, Asia – Poor Country Picture At the end of July 2010 usually heavy monsoon rains in northwest Pakistan caused rivers to flood and burst their banks. The map below shows the huge area of Pakistan affected by flooding. The floodwater slowly moved down the Indus River towards the sea.
Continuing heavy rain hampered the rescue efforts. After visiting Pakistan, the UN Secretary General, Ban Ki-moon, said that this disaster was worse than anything he’d ever seen. He described the floods as a slow-moving tsunami.
The effect of the floods · At least 1600 people died · 20 million Pakistanis were affected (over 10% of the population), 6 million needed food aid · Whole villages were swept away, and over 700,000 homes were damaged or destroyed · Hundreds of thousands of Pakistanis were displaced, and many suffered from malnutrition and a lack of clean water · 5000 miles of roads and railways were washed away, along with 1000 bridges · 160,000km2 of land were affected. That’s at least 20% of the country · About 6.5 million acres of crops were washed away in Punjab and Sindh provinces
The responses to the floods · Appeals were immediately launched by international organisation, like the UK’s Disasters Emergency Committee – and the UN – to help Pakistanis hit by the floods · Many charities and aid agencies provided help, including the Red Crescent and Medecins Sans Frontieres · Pakistan’s government also tried to raise money to help the huge number of people affected · But there were complaints that the Pakistan government was slow to respond to the crisis, and that it struggled to cope · Foreign Governments donated millions of dollars, and Saudi Arabia and the USA promised $600 million in flood aid. But many people felt that the richer foreign governments didn’t do enough to help · The UN’s World Food Programme provided crucial food aid. But, by November 2010, they were warning that they might have cut the amount of food handed out, because of a lack of donations from richer countries
- Students Projects
- Accomodation
- Career Services
- Financial Aid Services and Scholarships
- Guiding and Counselling
- Health Services
- International Students
- Online Learning
- PWD Services
- Student Organizations
- Academic Staff
- Technical Staff
- Administrative Staff
- Support Staff
- Register as Alumni
- Civil Engineering Facilities
- Facility Equipment
- Thesis Submission
- Achievements &Milestones
- Historical Photo Gallery
- Historical Projects
- Luminary Guests
- Staff Convocation List

looding is a major hazard affecting urban centres worldwide, including the city of Nairobi. This phenomenon is associated with loss of lives, destruction of property and disruption of the normal day to day activities. This study sought to identify the causes of these floods, focussing on the South C area of Nairobi which is prone to flooding. The study further sought to investigate the impact of flooding in the study area and examine the policy and legal strategies put in place to manage the menace of flooding in Nairobi County. Finally, the study proposed possible solutions to the flooding in the study area. In regard to finding out the causes of flooding, data from the meteorological department was analysed, maps of the existing drainage in the area were studied, questionnaires were issued to the residents of the area and past reports on the subject were studied. The major causes of the flooding in the area were found to be poor maintenance of the drainage systems, small capacity of the drainage system, vast areas of impervious surface and climate change. The area was also found to be located on a flood plain with the adjacent areas seated on higher altitudes. From this study it was noted that the major impact of flooding in the study area was disruption of the daily activities and discomfort of the residents. The residents found it difficult to access their work places and to take their children to school. Overflow of the storm runoff into the households caused discomfort and destruction of the household property. This study also ascertained that there are various legal, policy and institutional arrangement that have been put in place in order to combat the dangers posed by natural hazards in Kenya, However, the study determined that there are no specific policies or legal recommendations that have been formulated by the government towards mitigation of flooding hazards in urban centres. From this study, it was established that there is need for the relevant authorities to ensure that drainage infrastructure is regularly maintained. In addition to this, the drainage capacity of the channels should be increased so as to accommodate the runoff during storm events. This study also recommended that the residents in the area be sensitized to take part in cleanliness and maintenance of the drainage channels and their environment as a whole. Finally, the government should undertake to construct a dam or rehabilitate the existing Nairobi Dam so as to direct the storm waters into the dam for storage.
© University of Nairobi 2024. All rights Reserved.
Simulating the effect of rainwater harvesting on flood mitigation: the case of Asunción, Paraguay
- Original Paper
- Published: 23 October 2024
Cite this article
- Annette Thiessen-Anttila 1 ,
- Marcelo Castier 2 , 3 &
- Paula de Barros Barreto ORCID: orcid.org/0000-0001-8473-8296 4 an1
This work uses computer simulations to assess the effect of rainwater harvesting on the mitigation of flash floods in Asunción, Paraguay, where local rainfalls frequently cause damages, disruptions, and personal harm. Minute-by-minute rainfall data available for the year 2017 were used to select the storms for this study. Terrain, hydrographic, land cover, buildings information, and street maps were retrieved from various freely available sources. One of the hydrographic basins in the municipality of Asunción was chosen for analysis. Terrain and buildings data were merged so that the simulations predict how the built structures divert water flow. An effective rainfall equation was derived to account for the collective effect of thousands of rainwater harvesting systems (RWHS), which were assumed to be installed under different scenarios. The HEC-RAS software (Hydrologic Engineering Center River Analysis System, U.S. Army Corps of Engineers) was used. Three specific locations within the simulated basin were selected for a detailed study. Two peak flow rates were observed in most scenarios: the first of them is the immediate outcome of the rainfall and the second results from the downhill water flow. According to the simulations, the RWHS delay the largest peak flow rate by a few minutes (from 3 to 21 min, depending on the case), which might guide the possible deployment of early warning systems. In a scenario of RWHS with large tanks, installed on every roof – taken as the upper limit of what could be accomplished – reductions of up to 52.2% and 73.3% in the first and second peak flow rate, respectively, were calculated under a heavy rainfall. Under a moderate rainfall, these values were 45.5% and 78.1%. If it is considered that only 50% of the roof area is connected to RWHS, with smaller storage tanks, the peak flow rate reductions are of up to 17.7% (first peak) and 17.8% (second peak) under a heavy rainfall. Under a moderate rainfall, the corresponding values were 23.1% and 40.3%. The overall conclusion is that the effect of RWHS on flood mitigation, as measured by the peak flow rate, is highly dependent on the rain intensity, location, and RWHS features. A limitation of this study is its focus on a single water basin within Asunción’s metropolitan region. However, applying the established methodology to all water basins in a region of interest should provide information to support the decision-making process of policy makers at local and national levels.
This is a preview of subscription content, log in via an institution to check access.
Access this article
Subscribe and save.
- Get 10 units per month
- Download Article/Chapter or eBook
- 1 Unit = 1 Article or 1 Chapter
- Cancel anytime
Price includes VAT (Russian Federation)
Instant access to the full article PDF.
Rent this article via DeepDyve
Institutional subscriptions
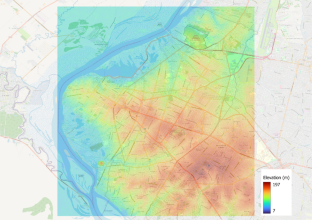
Availability of data and materials
the data files are available upon request.
Code availability
not applicable
ABC Color: Con helicópteros y buzos militares y policias buscan a desaparecidos en Lambaré. Accessed on November 6, 2023 (2023). https://www.abc.com.py/nacionales/2023/11/02/con-helicopteros-y-buzos-militares-y-policias-buscan-a-desaparecidos-en-lambare/
ABC Color: Se necesitan US\$ 200 millones para cubrir 100% de desagüe en Asunción. Accessed on July 17, 2024 (2019). https://www.abc.com.py/edicion-impresa/notas/se-necesitan-us-200-millones-para-cubrir-100-de-desage-en-asuncion-1813446.html
Abdelshafy M, Mostafa A (2021) Flash flood modeling using HEC-RAS (2D) model on Wadi Reem in the Western Region, Kingdom of Saudi Arabia. J Egypt Acad Soc Environ Develop 22(1):17–32
Article Google Scholar
Akter A, Tanim AH, Islam MK (2020) Possibilities of urban flood reduction through distributed-scale rainwater harvesting. Water Sci Eng 13(2):95–105
American Meteorological Society A (2012) Rain. Last edited on April 25, Accessed on November 13, 2023. https://glossary.ametsoc.org/wiki/Rain
Arquitectos del Paraguay: ¿Cómo se solucionan los problemas de inundaciones y raudales en Asunción y el AMA? Accessed on July 16, 2024 (2024). https://arquitectos.com.py/2024/04/como-se-solucionan-los-problemas-de-inundaciones-y-raudales-en-asuncion-y-el-ama/
Asunción M Ordenanzas Y Edictos. https://www.asuncion.gov.py/ordenanzas
Behzadian K, Kapelan Z, Mousavi SJ et al (2018) Can smart rainwater harvesting schemes result in the improved performance of integrated urban water systems? Environ Sci Pollut Res 25(19):19271–19282
Biswas BK, Mandal BH (2014) Construction and evaluation of rainwater harvesting system for domestic use in a remote and rural area of Khulna, Bangladesh. Int Schol Res Notices, 751952
Buta C, Mihai G, Stănescu M (2017) Flash floods simulation in a small drainage basin using HEC-RAS hydraulic model. Ovidius Univ Ann Ser Civil Eng 19:101–118
Google Scholar
DEFRA: Flood Risks to People, Phase 2,FD2321/TR2, Guidance Document. Technical report, Department for Environment, Food and Rural Affairs, Flood Management Division, London, UK (2006)
ESSAP and Municipality of Asunción: Desague Pluvial in Asunción. Accessed on August 2, 2024 (2016). https://geo-ide.carto.com/viz/4ccc0140-b33c-11e6-85b6-0e05a8b3e3d7/embed_map
Falcón-Araujo HA, Bellassai-Gauto MA, Barros Barreto AP, Nogués-Peña JP Variabilidad Espacial Y Temporal de la Lluvia (2018) https://hidrogeolab.upa.edu.py/variabilidad-espacial-y-temporal-de-la-lluvia/
Farreny R, Morales-Pinzón T, Guisasola A, Tayà C, Rieradevall J, Gabarrell X (2011) Roof selection for rainwater harvesting: quantity and quality assessments in Spain. Water Res 45(10):3245–3254
Article CAS Google Scholar
Ferreira MJ, Rocha HR (2023) Green roof infrastructure outperforms grey technology in flood mitigation in São Paulo’s urbanized region. Front Built Environ 9
Freni G, Liuzzo L (2019) Effectiveness of rainwater harvesting systems for flood reduction in residential urban areas. Water 11(7):1389
Grassi B, Pasten AM, Armoa J (2005) Un análisis del comportamiento de la precipitación en el Paraguay. Technical report, Facultad Politécnica, Universidad Nacional de Asunción
Hoy: Lluvias y tormentas: las calles y avenidas que deben evitarse en Asunción. Accessed on September 18, 2024 (2024). https://www.hoy.com.py/nacionales/2024/05/02/lluvias-y-tormentas-las-calles-y-avenidas-que-deben-evitarse-en-asuncion
Jamali B, Bach PM, Deletic A (2020) Rainwater harvesting for urban flood management–an integrated modelling framework. Water Res 171:115372
La Nación: Son once los puntos críticos en Asunción, advierten desde el municipio. Accessed on September 18, 2024 (2022). https://www.lanacion.com.py/pais/2022/03/23/son-once-los-puntos-criticos-en-asuncion-advierten-desde-el-municipio/
Nomad Season: Monthly climate in Asuncion, Paraguay. Accessed on June 5, 2023 (2022). https://nomadseason.com/climate/paraguay/asuncion/asuncion.html
Ortiz S, de Barros Barreto P, Castier M (2022) Rainwater harvesting for domestic applications: The case of Asunción. Paraguay. Results Eng 16:100638
Palla A, Gnecco I (2022) On the effectiveness of domestic rainwater harvesting systems to support urban flood resilience. Water Resour Manag Int J Publ Eur Water Resour Assoc (EWRA) 36(15):5897–5914
Peker IB, Gülbaz Sr, Demir V, Orhan O, Beden N (2024) Integration of HEC-RAS and HEC-HMS with GIS in flood modeling and flood hazard mapping. Sustainability 16(3):1226
Pereira B, Wehrle A, Pasten M (2021) Adaptación de la curva IDF de la ciudad de Asunción teniendo en cuenta los efectos del cambio climático. In: XXIV Simpósio Brasileiro de Recursos Hódricos (ISSN 2318-0358), Belo Horizonte, 21-26 November. Associação Brasileira de Recursos HÃdricos
Rangari VA, Umamahesh NV, Bhatt CM (2019) Assessment of inundation risk in urban floods using HEC RAS 2D. Model Earth Syst Environ 5(4):1839–1851
Rangari VA, Umamahesh NV, Patel AK (2021) Flood-hazard risk classification and mapping for urban catchment under different climate change scenarios: a case study of Hyderabad city. Urban Climate 36:100793
Razi MAM, Adnan MS, Abustan MSH, Uma MHA, Anuar ND, Jamal MH (2023) Flood modelling at Bandar Batu Pahat, Johor using HEC-RAS software. IOP Conf Ser Earth Environ Sci 1205:012023
Santos MM, Ferreira AV, Lanzinha JCG (2023) The possibilities of capturing rainwater and reducing the impact of floods: a proposal for the city of Beira, Mozambique. Sustainability 15(3):2276
Siphambe TV, Aliyu A, Souadji K, Bayongwa SA, Amans T, Fomena-Tchinda H, Banaon PY, Gina CS, Vuai HA, Farah AM, Niang AB, Taicha A, Ahmed S, Bashir A, Abdelbaki C, Mwamila TB, Gwenzi W, Nya EL, Noubactep C (2024) Mitigating flash flooding in the city: Drain or harvest? Water Supply 24(3):812–834
Soh QY, O’Dwyer E, Acha S, Shah N (2023) Robust optimisation of combined rainwater harvesting and flood mitigation systems. Water Res 245:120532
Thi LD, Van TD, Van HL (2020) Detection of flash-flood potential areas using watershed characteristics: Application to Cau River watershed in Vietnam. J Earth Syst Sci 129:120
Thiessen-Anttila A (2023) Impacto de los sistemas de captación de lluvia en raudales de una cuenca de asunción mediante simulaciones en HEC-RAS. Master’s thesis, German Paraguayan University, San Lorenzo, Paraguay
United Nations Habitat: Asunción Hazards and Challenges. Accessed on July 16, 2024. https://urbanresiliencehub.org/city-hazards/asuncion-es/
Última Hora: Unas 800 personas evacuadas y un muerto por fuerte temporal en Asunción. Accessed on April 3, 2024 (2014). https://www.ultimahora.com/unas-800-personas-evacuadas-y-un-muerto-fuerte-temporal-asuncion-n770670
Última Hora: Raudales en las principales avenidas de Asunción, un problema de nunca acabar. Accessed on September 18, 2024 (2022). https://www.ultimahora.com/raudales-las-principales-avenidas-asuncion-un-problema-nunca-acabar-n2992777
Wahab B, Ojolowo SK (2013) Rainwater harvesting: harnessing traditional approach to reduce flash floods in Ibadan metropolis. Ibadan Plann J 2(1):39–60
Wehrle A, Pereira B, Pasten M, Aróvalos F (2021) Estudios complementarios hidrolágicos e hidróulicos del Bañado Sur– Barrio Tacumbú, Producto V, Diseño Preliminar. Technical report, Ministerio de Obras Publicas y Comunicaciones, Paraguay
Download references
Acknowledgements
The authors thank Dr. Arnoud Cuppens for his valuable suggestions in the early stages of this work. The authors acknowledge the Consejo Nacional de Ciencia y Tecnología (CONACYT) of Paraguay for granting access to their CICCO bibliographic resources.
no funding was received for conducting this study.
Author information
Authors and affiliations.
Facultad de Ciencias de la Ingeniería, Universidad Paraguayo Alemana, Lope de Vega 1279, San Lorenzo, Paraguay
Annette Thiessen-Anttila
Texas A&M University at Qatar, Education City, Doha, Qatar
Marcelo Castier
Polytechnic Faculty, National University of Asunción, San Lorenzo, Paraguay
Department of Chemical Systems Engineering, School of Chemical Engineering, University of Campinas, Campinas, 13083-852, Brazil
- Paula de Barros Barreto
Author notes
Paula de barros barreto—work done, in part, while at universidad paraguayo alemana..
You can also search for this author in PubMed Google Scholar
Contributions
Annette Thiessen-Anttila: Methodology, Simulations, Formal analysis, Writing - Original Draft; Marcelo Castier: Conceptualization, Methodology, Simulations, Formal analysis, Writing - Review & Editing; Paula de Barros Barreto: Conceptualization, Methodology, Formal analysis, Writing - Review & Editing, Project management.
Corresponding author
Correspondence to Paula de Barros Barreto .
Ethics declarations
Conflict of interest.
the authors have no Conflict of interest to declare that are relevant to the content of this article.
Ethical approval
Consent to participate, consent for publication, additional information, publisher's note.
Springer Nature remains neutral with regard to jurisdictional claims in published maps and institutional affiliations.
Appendix A: Derivation of the effective rainfall formula
The amount of water harvested during a rainfall depends on the rain intensity and on the storage volume available because the harvesting tanks will collect rainwater until they fill up. To account for that, a time-dependent formula for the effective rainfall is derived. It is assumed that the hyetograph is known and that all its K time intervals are of equal duration. The amount of rain fallen between times \(t_{\ell -1}\) and \(t_{\ell }\) is represented by \(\Delta H_{\ell }\) (in mm or m of rain), where \(t_0\) is the time the rain begins to fall.
The maximum volume of rainwater that can be harvested during period \(\ell\) , \(\Delta {V_{h,\ell ,max}}\) , is:
where D is the average roof drainage coefficient, \(f_r\) is the fraction of the region surface that is covered by roofs, and a fraction \(f_c\) of the roof area is part of RWHS. The rightmost side of Eq. A1 embeds the definition of the area available for RWH:
With respect to storage, the symbol \(V_s\) represents the total storage capacity in the region, given by:
where \(\kappa\) is a specific storage capacity.
Writing \(V_s\) and \(A_{RWH}\) in terms of their average values per building, \({\overline{v} _s}\) and \({\overline{a} _{RWH}}\) , in a region with N buildings, it is found that:
As an example, if 5 m 3 is the average storage tank capacity and 200 m 2 is the average catchment area, we have that \(\kappa =0.025\) m 3 /m 2 . In other words, if such tank were initially empty, it would fill up when the amount of rain reached 0.025 m, that is, 25 mm.
When a rain episode begins, the storage tanks may be partially filled. The ability to catch water depends on the storage capacity initially available, \(V_{s,0}\) :
The storage capacity available is gradually reduced during the rain episode. At the end of a time period k , the storage volume still available is:
Dividing both sides of Eq. A6 by \({D{A_{RWH}}}\) , one obtains:
Water storage is possible until \(\frac{{{V_{s,k}}}}{{D{A_{RWH}}}}=0\) . In general, this condition would occur at a time inside some interval. For simplicity, k will be taken as the index of the interval with the smallest positive value of \(\frac{{{V_{s,k}}}}{{D{A_{RWH}}}}=0\) . In this way, an effective, reduced rain amount is calculated for the first k intervals; after that, the amount of rain is obtained directly from the hyetograph.
For each interval \(\ell\) , \(1 \le \ell \le k\) , the rain fallen and the rain harvested are, respectively, equal to:
During the same period, the amount of water that reaches the terrain is given by:
where Eq. A2 was used to eliminate \(A_{RWH}\) . This equation can be written in terms of an effective amount of rain fallen during interval \(\ell\) :
Canceling A on both sides of the equation, one obtains a formula for the effective rain fallen during interval \(\ell\) ( \(1 \le \ell \le k\) ):
Rights and permissions
Springer Nature or its licensor (e.g. a society or other partner) holds exclusive rights to this article under a publishing agreement with the author(s) or other rightsholder(s); author self-archiving of the accepted manuscript version of this article is solely governed by the terms of such publishing agreement and applicable law.
Reprints and permissions
About this article
Thiessen-Anttila, A., Castier, M. & de Barros Barreto, P. Simulating the effect of rainwater harvesting on flood mitigation: the case of Asunción, Paraguay. Nat Hazards (2024). https://doi.org/10.1007/s11069-024-06961-y
Download citation
Received : 11 April 2024
Accepted : 09 October 2024
Published : 23 October 2024
DOI : https://doi.org/10.1007/s11069-024-06961-y
Share this article
Anyone you share the following link with will be able to read this content:
Sorry, a shareable link is not currently available for this article.
Provided by the Springer Nature SharedIt content-sharing initiative
- Find a journal
- Publish with us
- Track your research
- 0 Shopping Cart £ 0.00 -->
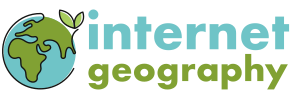
Tewkesbury Floods 2007 Case Study
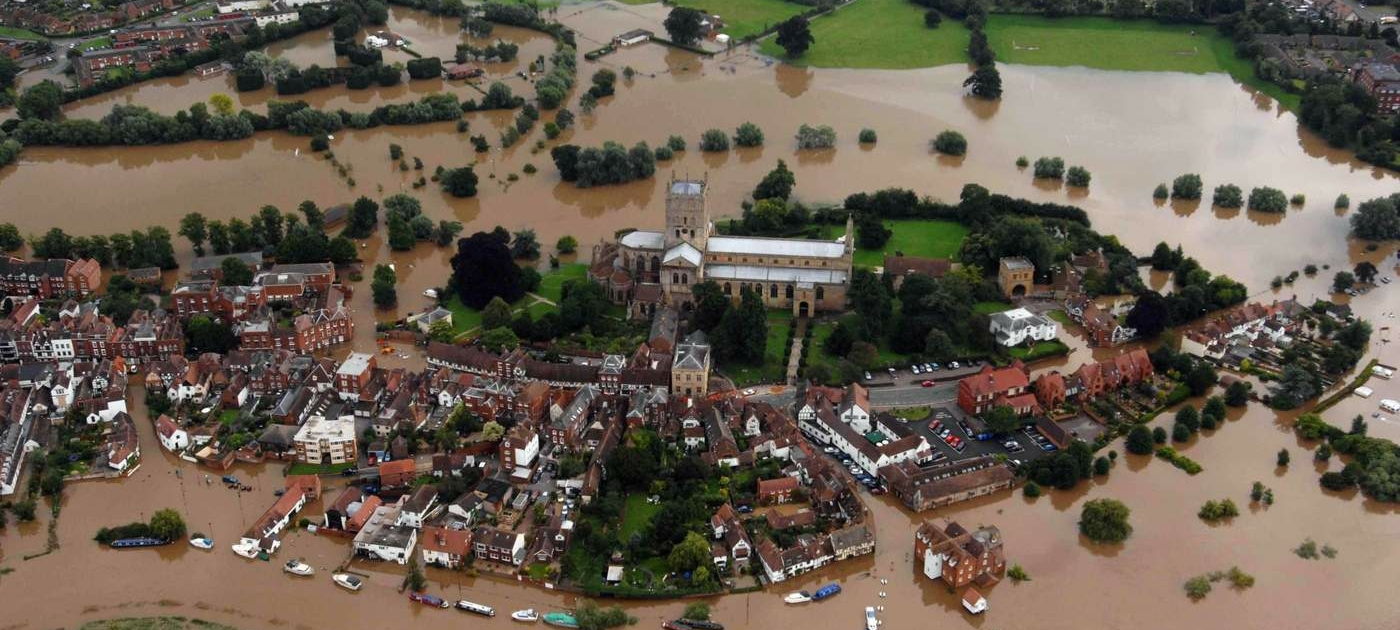
Home > Geotopics > Tewkesbury Floods 2007 Case Study
The historic town of Tewkesbury in Gloucestershire, UK, faced a catastrophic flooding event in July 2007. This incident, part of a broader pattern of severe floods across the UK during that summer, offers a vital case study for understanding the dynamics of flooding, particularly the intertwining of natural and human-induced factors.
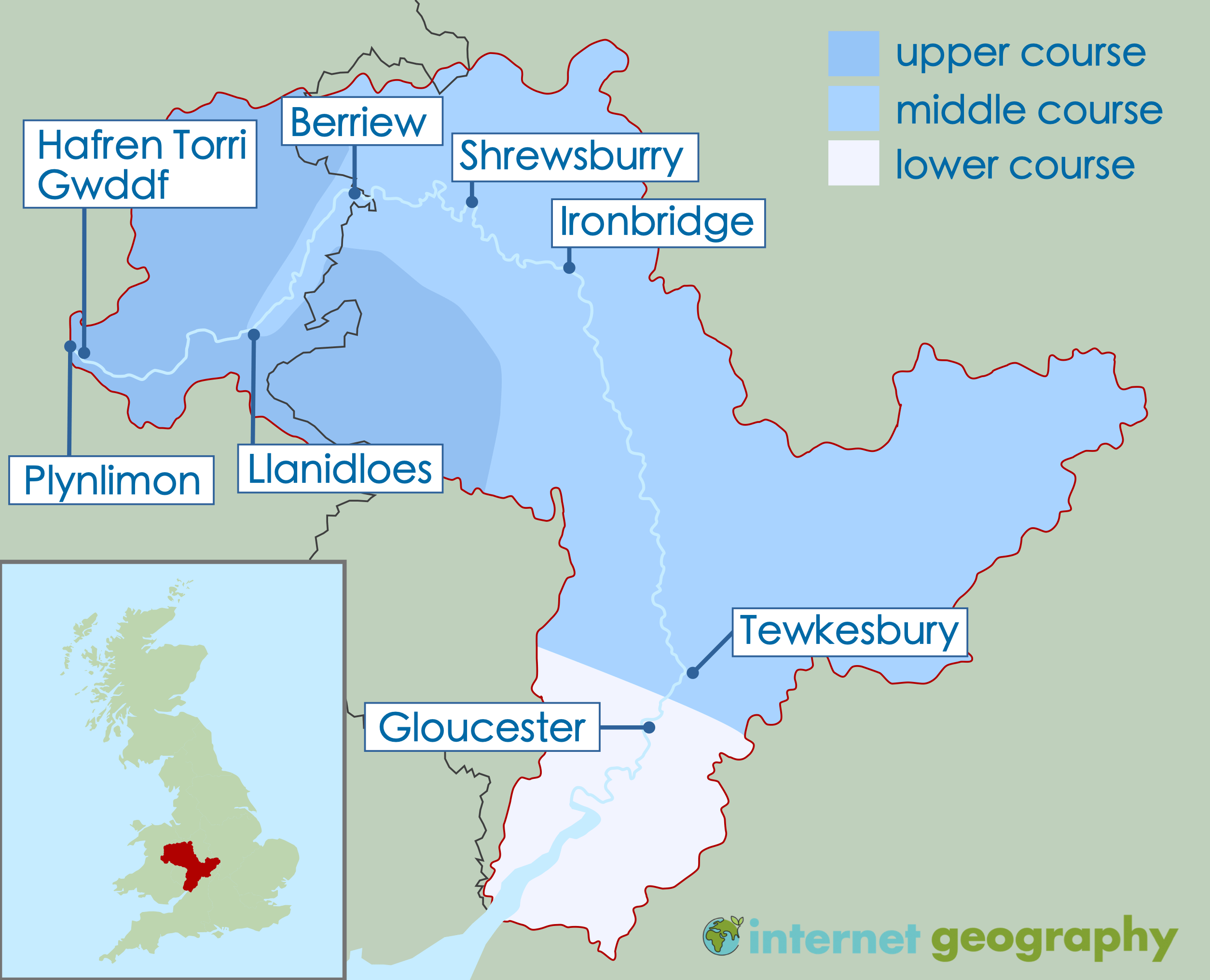
Tewkesbury on the River Severn
The Causes of the 2007 Tewkesbury Floods
The flooding in Tewkesbury was the result of both natural and human factors. The primary natural cause was the extreme and persistent rainfall during the summer, which led to the rivers Severn and Avon converging near Tewkesbury, overflowing their banks. The town’s geographical setting made it inherently susceptible to flooding. Additionally, the surrounding hills accelerated the run-off process, leading to an even greater influx of water into the river systems.
On the human side, the increased urban development in Tewkesbury and its surrounding areas contributed significantly to the flooding. Expanding impermeable surfaces like roads and buildings meant less rainwater could be absorbed into the ground, increasing the volume of run-off. Furthermore, the existing flood defence mechanisms were inadequate for such an extraordinary event. Changes in land use, including agricultural practices in the catchment area, also altered the natural water absorption and drainage patterns.
The Impacts of the Flood
The social impacts of the Tewkesbury floods were profound and multifaceted. Thousands of residents were displaced as over 3,500 homes were evacuated. The health risks posed by the floodwaters were significant, including threats of waterborne diseases and limited access to healthcare facilities due to the inundated infrastructure. The community faced considerable disruption, with schools closing down and local events being cancelled, affecting the town’s social fabric.
Economically, the floods inflicted substantial damage. The cost of damages to properties and infrastructure amounted to millions of pounds, heavily straining financial resources. Local businesses, especially those reliant on tourism , faced severe interruptions, leading to significant economic losses. The flood’s aftermath saw a surge in insurance claims and a need for considerable investment in reconstruction and recovery efforts.
Environmentally, the floods had far-reaching impacts. The local ecosystems experienced significant disruption, affecting both wildlife and plant life. Water pollution levels increased, with run-off from agricultural lands and overflowing sewage systems contaminating the waterways. The severity of the flooding potentially led to long-term changes in the landscape , including alterations in the courses of local rivers.
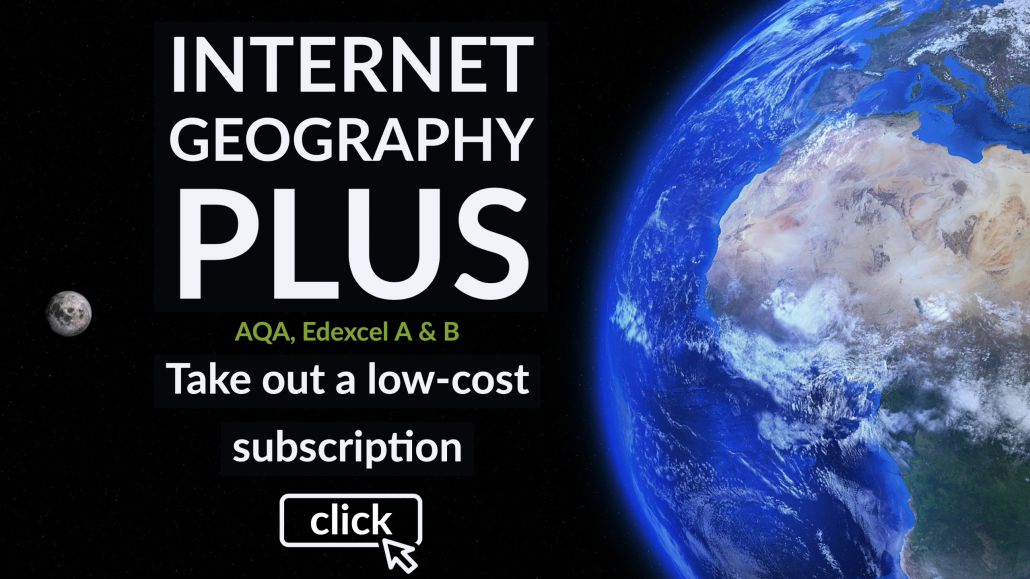
Premium Resources
Please support internet geography.
If you've found the resources on this page useful please consider making a secure donation via PayPal to support the development of the site. The site is self-funded and your support is really appreciated.
Related Topics
Use the images below to explore related GeoTopics.
Topic Home
Next topic page, share this:.
- Click to share on Twitter (Opens in new window)
- Click to share on Facebook (Opens in new window)
- Click to share on Pinterest (Opens in new window)
- Click to email a link to a friend (Opens in new window)
- Click to share on WhatsApp (Opens in new window)
- Click to print (Opens in new window)
If you've found the resources on this site useful please consider making a secure donation via PayPal to support the development of the site. The site is self-funded and your support is really appreciated.
Search Internet Geography

Latest Blog Entries

Pin It on Pinterest
- Click to share
- Print Friendly

COMMENTS
Effects. In some areas, floodwater was between 3-4.5m deep. Floods in the southern Indian state of Kerala have killed more than 410 people since June 2018 in what local officials said was the worst flooding in 100 years. Many of those who died had been crushed under debris caused by landslides.
The Somerset Levels Flood Case Study. The Somerset Levels are a coastal plain and wetland area in Somerset, England. Thousands of years ago, the area was covered by the sea, but today it's a landscape of rivers and wetlands - artificially drained, irrigated and modified to allow productive farming.. It is claimed that the Somerset Levels are one of the lowest areas in the UK.
The Missouri River and North Central Flood were the result of a powerful storm that occurred near the end of the wettest 12-month period on record in the U.S. (May 2018 - May 2019). CS_55, CS_56 The storm struck numerous states, specifically Nebraska (see Figure 1), Iowa, Missouri, South Dakota, North Dakota, Minnesota, Wisconsin, and Michigan.
3.16 Case Study - Flooding in Somerset (2013-2014) For a period of three months from December 2013 to February 2014, the Somerset Levels hit the national (United Kingdom) headlines as the area suffered from extensive flooding. At the height of the winter floods, 65 km2 of land on the Levels were under water.
Abstract and Figures. Floods are among disasters that cause widespread destruction to human lives, properties and the environment every year and occur at different places with varied scales across ...
Across case studies where two similar floods occurred in the same region, with the second flood causing substantially lower damage 15, the damage reduction is mainly attributed to substantial ...
Urban flooding has become a national challenge in recent years due to a variety of socio-economic and environmental changes alongside rapid land use change in flood-prone areas. Losses from acute and chronic floods have become especially problematic in low-lying urban areas, where stormwater infrastructure deterioration, population growth, and ...
Climate Change is Exacerbating the Effects of Floods. Flooding has been made more likely by climate change, according to a 2021 study by climate experts. In the case of Western Europe, downpours in the region, which caused the flash floods that killed nearly 200 people, are now 3-19% heavier due to human-caused warming.
The Mississippi river drains an area of nearly 3 million square kilometres and is the fifth largest river basin in the world. It provides a good case study as there are a range of human and physical causes, huge impacts of flooding and some good examples of effective action taken as a result. The river floodplain is up to 200km wide in the ...
As a result, the soil becomes loose and is susceptible to erosion. This causes more soil and silt in rivers. This leads to flooding in Bangladesh. Flooding is a significant problem in the Ganges and Brahmaputra river basin. They cause large scale problems in the low lying country of Bangladesh. There are both human and natural causes of ...
A reckless experiment in Earth's atmosphere caused a desert metropolis to flood. That was the story last week when more than a year's worth of rain fell in a day on the Arabian Peninsula, one ...
Through a comparison with generally accepted urban flooding management practices, we (the authors) identified that a lack of surface water runoff management considerations (i.e., missing a major drainage system in urban stormwater system) and inadequate local mitigation are the primary causes of urban flooding problems in China, which in turn ...
This study aimed to investigate flood incidence causes in the Ribb river. Possible causes of flooding were identified from literature. To recognize the causes, trend analysis of rainfall to know possible climate change, stream flow analysis to know possible land cover change and lake water level trend analysis to know backwater effect were ...
In 2019 severe monsoon rainfall in India led to flooding and over 1,600 deaths. There was 10% more rain than the 50 year average. Leads to the saturation of the soil. This means that rainfall cannot infiltrate. Increases surface run off which means the water reaches the rivers more rapidly increasing the risk of flood.
The effects of the flood. · Over 1300 homes were flooded and contaminated with sewage. · A number of people had to be evacuated, including 50 by helicopter, when the flooding cut off Cockermouth town centre. · Many businesses were flooded causing long-term difficulties for the local economy. · People were told that they were unlikely to be ...
The major causes of the flooding in the area were found to be poor maintenance of the drainage systems, small capacity of the drainage system, vast areas of impervious surface and climate change. The area was also found to be located on a flood plain with the adjacent areas seated on higher altitudes. From this study it was noted that the major ...
UK Floods Case Study November 2019 The UK experienced an extreme weather event in November 2019 when exceptionally heavy rainfall caused flooding in parts of the UK. Heavy downpours across large parts of northern England led to surface water and river flooding in parts of Yorkshire, Nottinghamshire, Greater Manchester, Derbyshire and Lincolnshire.
This work uses computer simulations to assess the effect of rainwater harvesting on the mitigation of flash floods in Asunción, Paraguay, where local rainfalls frequently cause damages, disruptions, and personal harm. Minute-by-minute rainfall data available for the year 2017 were used to select the storms for this study. Terrain, hydrographic, land cover, buildings information, and street ...
The flooding in Tewkesbury was the result of both natural and human factors. The primary natural cause was the extreme and persistent rainfall during the summer, which led to the rivers Severn and Avon converging near Tewkesbury, overflowing their banks. The town's geographical setting made it inherently susceptible to flooding.