Virtual Reality in Education: Benefits, Uses and Examples
Education is driving the future of VR more than any other industry outside of gaming. Here's why virtual reality gets such high marks for tutoring, STEM development, field trips and distance education.

Virtual reality in education may sound like science fiction, but these two industries go hand in hand better than you’d think. The growing field of VR has potential to enhance learning by providing students with access to virtual environments where they can engage with immersive content from a range of subjects, such as art, geography, biology and chemistry.
Read More A Guide to the Metaverse and VR Tech: What You Need to Know

What Is Virtual Reality in Education?
Virtual reality in education can be used in K-12 classrooms, for vocational training and in higher education settings. Since virtual reality allows users to interact with computer-simulated environments, it can enable virtual field trips, immerse students in historically significant events, simulate laboratory environments and build meaningful connections among instructors and peers despite the distance between them.
The virtual reality market size is expected to grow from less than $12 billion in 2022 to more than $22 billion by 2025, according to data from Statista . One of the factors motivating growth in the VR industry has been the demand for solutions to combat feelings of isolation during virtual, distanced learning.
VR classrooms have been able to give students opportunities to raise their hands, ask questions in an organic way and generally feel more directly invested. That’s in comparison to what CEO Mat Chacon of VR company Doghead Simulations described as the "pretty flat experience" of traditional online courses.
Doghead co-founder Chance Glasco said he had “no doubt” that online classes will one day be replaced by virtual reality.
“No one builds memories of online classes,” he told Built In in 2019. “It’s just data being fed to your brain in the most boring way possible.”
A 2022 National Research Group report on VR technologies revealed just over 60 percent of consumers who participated in the study “think that VR and AR will be a useful learning tool for children.” Another poll indicated 67 percent of U.S. high school educators surveyed said they want to see extended reality technologies like VR used regularly in schools. The majority of those teachers said the technologies have the potential to help students develop career skills, build social and empathy skills and stay more engaged and motivated in the classroom.
How Does Virtual reality in Education Work?
Virtual reality in education often involves viewing or interacting with learning content using a VR headset along with any associated hardware, such as controllers that can let the user navigate and manipulate a simulated reality. VR headsets use screens, lenses and other advanced technology like sensors that are designed to wrap the viewer in a 360-degree view of a virtual setting.
Some companies produce VR learning content that can be accessed on a desktop, laptop or tablet. In those cases, the content is not fully immersive, but students are still able to participate in simulated environments without the extra costs that can come with VR headsets, which can be a barrier to adoption.
While the science is still out on whether VR is more effective than other immersive-media learning tools, it appears to hold real pedagogical promise. A study by Stanford researchers looked at VR field trips about climate change and found that “participants who explored more of the virtual space formed deeper cognitive associations with the science content and could learn, recall and retain the causes and effects of ocean acidification better than those who did not explore the underwater world as much.”
Benefits of Virtual Reality in Education
Virtual reality has capabilities that could turn it into a valuable asset for education. For example, research out of Penn State University showed that students who used immersive virtual reality to accomplish a task did so more than twice as fast as students who used traditional computer programs.
Social VR applications like rumii from Doghead can also help tackle the challenge of sky-high dropout rates for online courses by helping remote students feel more connected and less isolated. Doghead partnered with Full Sail University to deploy rumii in online coursework to “make students and professors feel like they are in the classroom together.”
Rumii has also been used to facilitate collaboration among students on different continents. A group of anthropology students — half studying at Harvard University and half at Zhejiang University in China — were able to work together as avatars in a VR-equipped classroom to study ancient characters scrawled along a tomb atop the Giza Plateau in preparation for a trip to Egypt. The students were strapped into VR headsets as their professors launched the lab and loaded up 3D models of the Sphinx and one of the tombs, which the teams could then grab and move around in the virtual learning space. Other features of the experience included live HD video streaming and screen sharing.
“It was just this natural conversational immersive interaction that made their trip to Egypt a lot more valuable because, when they were there, they could hit the ground running,” Chacon explained.
Another VR advantage is the comforting semi-anonymity that avatars afford. There’s reams of research about the so-called Proteus Effect, or how a virtual reality user's behavior might be subtly affected by their avatar's characteristics. But Doghead believes those alterations have been positive in rumii.
“You get the comfort of being in person with someone because you feel present with them,” Glasco said. “But you feel safe behind a VR headset, behind this avatar, which represents your body language and your audio.”
How Virtual Reality Is Used in Education and Schools
Virtual field trips.
Discovery Education has reached millions of students with its virtual field trips , focusing on aerospace (a virtual behind-the-scenes tour of the Johnson Space Center), health (a VR-powered look at the science behind opioid addiction), technology (a multi-part series on agtech) and more. Along with Google Arts & Culture Expeditions — a VR app with more than a thousand educational tours — it's one of the leading distributors of educational VR field trips.
Art Education
“Blue-Fall,” a 1966 painting by Abstract Expressionism pioneer Helen Frankenthaler, is housed in the Milwaukee Art Museum’s permanent collection, but you don’t have to trek to Wisconsin to experience it. Any VR user can virtually zoom in on Frankenthaler’s bold, cobalt monolith — and even listen to author Neil Gaiman play docent as he lends art-historical context and detail.
Gaiman is an advisory board member of Boulevard , a New York-based art-education VR company that brings the museum and gallery experience to virtual reality. Experiences range from a survey of pre-Raphaelite painter Dante Gabriel Rossetti to a sample of Turner Prize winner Grayson Perry’s 2015 solo exhibition. Another notable example of the growing virtualization of the art experience is The Kremer Museum , which arranges 74 paintings of Dutch and Flemish Old Masters in a virtual gallery.
A Virtual Lab Environment
Between 2021 and 2031, the number of STEM occupations in the United States will grow by nearly 11 percent in comparison to less than 6 percent for all other jobs, according to forecasts from the U.S. Bureau of Labor Statistics .
But state-of-the-art labs where so much hands-on STEM learning takes place can be difficult and costly to access. Labster democratizes the process with virtual lab environments for more than two dozen course packages , including high school physics, biosciences for nursing, animal physiology, advanced biology and engineering. The labs — which can be accessed via a web browser without downloading or installing additional software — allow students to culture bacteria, track cellular respiration during an exercise routine, even conduct an ultrasound exam on an expectant mother — virtually speaking, of course. Labster also owns UbiSim , a VR training platform for nurses that lets learners participate in risk-free clinical scenarios using VR headsets and controllers.
Workforce Training
Lifeliqe develops immersive simulations for workforce training, preparing professionals for in-demand careers in fields like healthcare and advanced manufacturing. The company’s programs involve exploration of the work environment and responsibilities, VR models of necessary tools, training simulations and assessment’s to aid in tracking student progress. The current product offerings from Lifeliqe include courses for dialysis technicians and certified nursing technicians, but the company also has training programs in the works for manufacturing and HVAC technicians.
Further Reading Everything You Need to Know About Extended Reality
The Future of Virtual Reality in Education
Despite virtual reality’s ever-widening footprint in the education sector, some challenges persist. Pre-undergraduate education isn’t exactly flush with dollars, so it can be difficult for forward-thinking startups to get a proverbial foot in the door.
“When you’re dealing with education, especially K-12, funds are limited,” Glasco said. “You have to get to buyers at the right time, or you might be talking to them for a year before they sign on to a license. There is money in education; you just have to stick around long enough to be able to tap into it.”
And even though the technology is advanced enough to be a powerful educational tool, some experts say improved curriculum development is key to making VR an appreciably more effective tool than interactive 2D content.
The XR Association and International Society for Technology in Education’s survey of more than 1,400 U.S. high school teachers on their attitudes toward extended-reality technologies like VR showed that the majority believe virtual learning experiences provide quality information. Yet more than half also see the costs associated with these technologies as having the potential to widen equity gaps. And 94 percent agreed curriculum associated with technology such as VR needs to be aligned with academic standards.
"I think the developer community and the education community need to walk down this road very hand-in-hand," Chacon said. "Then we can start bridging social classes and removing all of these barriers to education."
He noted that leaps in digital lightfield technology are steering virtual reality toward a distinctly Holodeck -like future — no wearables required.
“It seems like it's really far in the future,” Chacon said, “but it's already happening.”
Recent Edtech Articles
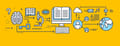
Advertisement
Immersive virtual reality as a pedagogical tool in education: a systematic literature review of quantitative learning outcomes and experimental design
- Open access
- Published: 11 July 2020
- Volume 8 , pages 1–32, ( 2021 )
Cite this article
You have full access to this open access article
- D. Hamilton ORCID: orcid.org/0000-0002-8659-4385 1 ,
- J. McKechnie 1 ,
- E. Edgerton ORCID: orcid.org/0000-0001-7389-527X 1 &
- C. Wilson ORCID: orcid.org/0000-0003-1054-4928 1
82k Accesses
353 Citations
71 Altmetric
Explore all metrics
The adoption of immersive virtual reality (I-VR) as a pedagogical method in education has challenged the conceptual definition of what constitutes a learning environment. High fidelity graphics and immersive content using head-mounted-displays (HMD) have allowed students to explore complex subjects in a way that traditional teaching methods cannot. Despite this, research focusing on learning outcomes, intervention characteristics, and assessment measures associated with I-VR use has been sparse. To explore this, the current systematic review examined experimental studies published since 2013, where quantitative learning outcomes using HMD based I-VR were compared with less immersive pedagogical methods such as desktop computers and slideshows. A literature search yielded 29 publications that were deemed suitable for inclusion. Included papers were quality assessed using the Medical Education Research Study Quality Instrument (MERSQI). Most studies found a significant advantage of utilising I-VR in education, whilst a smaller number found no significant differences in attainment level regardless of whether I-VR or non-immersive methods were utilised. Only two studies found clear detrimental effects of using I-VR. However, most studies used short interventions, did not examine information retention, and were focused mainly on the teaching of scientific topics such as biology or physics. In addition, the MERSQI showed that the methods used to evaluate learning outcomes are often inadequate and this may affect the interpretation of I-VR’s utility. The review highlights that a rigorous methodological approach through the identification of appropriate assessment measures, intervention characteristics, and learning outcomes is essential to understanding the potential of I-VR as a pedagogical method.
Similar content being viewed by others
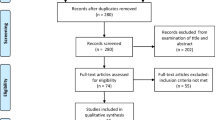
Impact of immersive realities and metaverse in the university training for health professions and medicine: a systematic review of preliminary evidence

Immersive Virtual Reality in K-12 and Higher Education: A systematic review of the last decade scientific literature
Educational Opportunities for Immersive Virtual Reality
Avoid common mistakes on your manuscript.
Introduction
The increasing financial feasibility of virtual reality (VR) has allowed for educational institutions to incorporate the technology into their teaching. According to research, 96% of universities and 79% of colleges in the UK are now utilising augmented or virtual reality in some capacity (UKAuthority 2019 ). In addition, the rising power of personal computers and associated hardware has led to a revolution in graphical fidelity, with ever more complex and realistic simulations and virtual worlds (Slater 2018 ). As Dickey ( 2005 ) alludes to, this has both challenged and expanded the very conceptual definition of what is defined as a learning environment. Where once this would have been restricted to classroom teaching or field trips, VR’s innate ability to give users a sense of presence and immersion has opened new possibilities in education if implemented appropriately (Häfner et al. 2018 ).
The use of technology-aided education as a pedagogical method is not a modern phenomenon, and investigations into its utility have been studied for almost half a century. As far back as the 1970s, Ellinger and Frankland ( 1976 ) found that the use of early computers to teach economic principles produced comparative learning outcomes with traditional didactic methods such as lectures. However, as Jensen and Konradsen ( 2018 ) allude to, it was with the release of the Oculus Rift in 2013 that VR became synonymous with head-mounted-display (HMD) based VR. This had several ramifications. First, HMDs became economically feasible for consumers and educational institutions to acquire en masse , due to a significant drop in price (Hodgson et al. 2015 ). As Olmos et al. ( 2018 ) remarks, the economic viability of VR has tackled one of the main entry barriers to adopting the technology. And secondly, academic research into the potential benefits of I-VR in education starts to expand, as well as its applied use in pedagogical settings (Hodgson et al. 2019 ). One of VR’s most important contributions to education is that it has allowed students to repeatedly practice complex and demanding tasks in a safe environment. This is particularly true of procedural tasks such as surgical operations or dental procedures that cannot be carried out for real until a certain level of competency has been achieved (Alaraj et al. 2011 ; Larsen et al. 2012 ). Additionally, VR has allowed for students to gain cognitive skills by way of experiential learning, such as exposing them to environments that would be too logistically problematic to visit in reality (Çalişkan 2011 ). For instance, by using a HMD, Bailenson et al. ( 2018 ) were able to expose students to an underwater environment to facilitate learning about climate change. VR has made an important contribution to education in that it has allowed for students to directly experience environments or situations that are difficult to replicate by using traditional teaching methods such as lectures, slideshows, or 2D videos.
A concise definition of VR’s key characteristics is challenging due to the ever-changing nature of the technology. However, Sherman and Craig ( 2003 ) proposed that there are a number of constituent elements that must underpin the VR experience, ultimately leading to the life-like perception of the virtual environment. These include the necessity for VR to be immersive, in that the participant’s own cognitive faculties produce a sense of being present and involved in the virtual space, often with reduced awareness of what is happening in the real-world around them. Additionally, the virtual space should offer a degree of interactivity, in that the user can manipulate the environment and test variables. This can include interacting with objects, virtual avatars, or even collaborating with other real-life users within the computer-generated space.
Definition of key terms
Due to the multidisciplinary nature of VR research and its pedagogical applications, it is important to define key terms used. VR can broadly be broken down into two main categories: desktop VR (D-VR), and immersive-VR (I-VR). D-VR is typically classified as non-immersive, in that a headset is not used, and the participant will be controlling and manipulating the virtual environment on a computer screen with traditional keyboard and mouse hardware (Lee et al. 2010 ). On the other hand, I-VR is typically multi-modal in nature by providing a sense of immersion in the environment through 360° visuals by aid of a HMD, auditory stimulation through the use of earphones, and increasingly the proprioception of limbs by way of controllers and tracking (Freina and Ott 2015 ; Howard-Jones et al. 2015 ; Murcia-López and Steed 2016 ). Although there are a range of HMDs on the market, from high-end hardware like the HTC Vive, to viable low-cost options like the Google Cardboard, they all utilise the same core principals of operation (Brown and Green 2016 ). Typically, a HMD will feature a set of embedded liquid crystal displays (LCD) which will present each eye an image from a slightly different angle. This mimics natural optic function by allowing the wearer to view a stereoscopic image complete with depth perception and a wide field of view. Mobile VR headsets can achieve the same effect using a single display by dividing the screen down the middle and presenting each half to the corresponding eye. Therefore, the current review defines a HMD as a device worn over the head, which provides a stereoscopic computer-generated or 360 ° video image to the user. This includes tethered (connected to a computer), stand-alone (no computer needed), or mobile VR headsets (mobile/cell phone connected to a HMD).
Previous literature and reviews
There have been a number of systematic reviews that have previously explored the relationship between VR and pedagogical attainment. Lee ( 1999 ) reviewed 19 studies from as far back as 1976 and found that 66% of students in simulation groups outperformed those in their respective control groups. However, this review did not focus exclusively on an educational level or age range, so featured both young kindergarten children, as well as higher education students. As a result, the generalisability of VR’s effectiveness as a pedagogical method is difficult to ascertain, with significant differences in age, task difficulty, and applications. Furthermore, all the studies are dated in terms of the technology utilised and feature early D-VR programmes and rudimental computer simulations. This early technology may be primitive when compared with the high-fidelity graphics and immersive components of contemporary technology. Nevertheless, these early studies do help exemplify that the use of technology in education is not a new concept, and computer-based simulations have long been employed as a way of facilitating learning.
A more recent analysis was undertaken by Merchant et al. ( 2014 ), and looked at three specific sub-categories of VR: games, simulations, and virtual worlds. Games give the actor autonomy and freedom to move around the virtual world, testing hypotheses, achieving goals, and eliciting motivation and learning through immersion (Gee 2004 ). Simulations attempt to recreate a real-world environment that can help facilitate learning by allowing for the testing of variables and resulting outcomes. Finally, virtual worlds can provide an immersive or non-immersive sense of presence in a three-dimensional (3D) world, and the ability to manipulate, interact, or construct objects. Furthermore, virtual worlds can give the opportunity for multiple users to interact with one another within the digital environment (Dickey 2005 ). The meta-analysis showed that although game-based VR produced the highest learning outcomes, simulations and virtual worlds were also effective at increasing educational attainment. Once again, the limitation of this review is that it did not restrict its analysis to exclusively one domain of education. Although higher education made up the greatest number of studies, research from elementary and middle school were also included in the analysis.
One of the most recent systematic reviews to look exclusively at I-VR through the utilisation of HMDs was carried out by Jensen and Konradsen ( 2018 ). In their comprehensive search of existing literature published between 2013 and 2017, the review identified 21 quantitative and qualitative papers that focused on both learning outcomes in I-VR, and subjective attitudes and experiences on the part of the user. The review found limited effectiveness of HMD in the acquisition of cognitive, psychomotor, and affective skills when compared with less immersive technologies. However, Jensen and Konradsen ( 2018 ) did highlight the relatively low quality of studies included as a concern, and this may impede the ability to draw firm conclusions about the educational utility of I-VR.
Rationale for review
There are several fundamental reasons that necessitate an updated assessment of the topic area, such as the increase in relevant published literature, as well as the narrow scope of previous reviews. The last major review looking at I-VR and HMDs as an educational tool was carried out by Jensen and Konradsen ( 2018 ), with the most recent studies featured in that paper being published in 2016. Since then, there has been a significant increase in relevant published literature, with > 70% of the papers included in the current review being published since 2017, and therefore not included in the previous systematic review. Additionally, unlike previous reviews, the current examination of I-VR’s pedagogical utility focuses exclusively on studies where I-VR is directly compared to a less immersive method of learning. As a result, the current paper is able to highlight not only whether I-VR is an effective medium, but also whether it is more effective when compared to alternative methods. Additionally, no other systematic review looking at I-VR and HMDs has had a particular focus on the experimental design, assessment measures, and intervention characteristics of the included studies. The review also addresses the underlying methodology of the included studies, to offer an understanding of how I-VR is being employed in experimental literature. Based upon the findings of previous studies as well as areas yet to be sufficiently explored, this paper has a number of core research questions:
To assess the subject area, discipline, and learning domain that I-VR has been employed in.
Understand where I-VR confers an educational benefit in terms of quantitative learning outcomes over non-immersive and traditional teaching methods.
To examine the experimental design of studies, focusing on how learning outcomes are assessed, and how the I-VR intervention is delivered.
To inform future experimental and applied practice in the field of pedagogical I-VR application.
Methodology
Search strategy.
The current systematic review included peer-reviewed journal articles and conference proceedings that passed all the inclusion criteria detailed. An initial scoping review identified seven databases that could be utilised in a comprehensive literature review, as well as associated keywords and search terms. These included Web of Science (Core Collection), Science Direct, Sage, IEEE Xplore, EBSCO, Taylor & Francis, and Google Scholar. These databases encompass a mixture of general, social science, and technological literature.
Each of the seven databases was searched using a series of keywords based on the following Boolean logic string:
("Virtual Reality" OR "Virtual-Reality" OR “Immersive Virtual Reality” OR “Head Mounted Display” OR “Immersive Simulation”) AND (Education OR Training OR Learning OR Teaching)
Due to the scope and parameters of the research objectives, only peer-reviewed literature published between January 2013 and December 2018 was included in the final review. Early access articles due to be published in 2019 were also included if these were found using the database searches. Date criteria was based upon an initial scoping review that found a substantial growth in relevant I-VR literature from 2013 onwards. A major contributing factor was the release of the Oculus Rift Development Kit 1 (DK-1) in early 2013, which is regarded as one of the first economically viable and high quality HMDs that could be used both within educational institutions, and at home (Lyne 2013 ).
The literature search across the databases yielded more than 12,000 references from a variety of sources. After the removal of duplicate records, 9,359 unique references were included for the title and abstract screening stage of the review.
Selection and screening
The open and general nature of the search string used led to a large number of references being returned for screening. As Jensen and Konradsen ( 2018 ) already alluded to in the last major review, VR research transcends various academic disciplines. The result is a lack of a clear taxonomy of definitions and terms. This means a wide net must be cast to ensure comprehensive capture of relevant material. This review defined I-VR as either a completely computer-generated environment, or the viewing of captured 360 ° video through the use of a HMD. Studies that utilised surgical or dental simulators and trainers such as the da Vinci Surgical System, were excluded as these represent a separate domain of both technological and pedagogical application. For example, surgical simulation based VR typically combines computer-generated visuals with simulated surgical tools, haptic feedback, and robotic components (Li et al. 2017 ). This type of technology would therefore not be applicable for general pedagogical application. Additionally, references were excluded if they: (1) focused on using I-VR as a rehabilitation or therapeutic tool; (2) were not in English; or (3) where the full-text was not available.
After title and abstract screening was performed, 197 references remained to be included in the full-text review. Each reference had to pass an inclusion flowchart based on each of the following criteria:
The population being sampled was from a high school, further or higher education establishment, or was an adult education student.
Population sampled did not have a developmental or neurological condition, nor could VR be used as a rehabilitation tool.
Paper described an experimental or quasi-experimental trial with at least one control group.
At least one group had to have undergone an educational HMD I-VR experience, and was compared with another group who underwent a non-immersive or traditional pedagogical method of education (e.g. Desktop VR, PowerPoint, traditional lecture).
A quantitative and objective learning outcome such as tests scores, completion time, or knowledge retention was used to assess effectiveness.
After full-text screening, 29 references passed all stages and were included in the systematic review. See Fig. 1 for a summary of the selection process by stage.
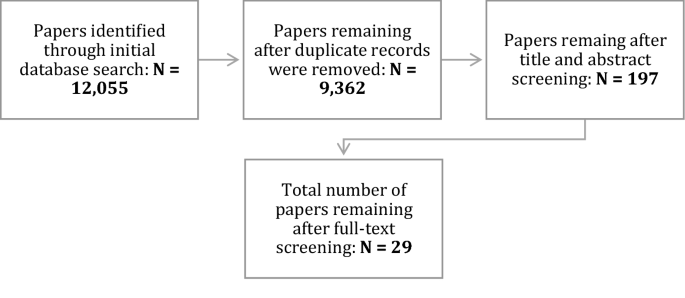
Stage-by-stage selection process
Inter-rater reliability checks were conducted at the title and abstract screening stage to assess the agreement of included studies. There were four individual evaluators that assessed the suitability of each reference based upon the inclusion criteria, which yielded an average agreement of 96%. Where any disagreement existed, the paper was discussed among all assessors until a unanimous decision was reached as to its suitability.
Quality assessment tool
To assess the quality of the studies, the Medical Education Research Study Quality Instrument (MERSQI) was used (Reed et al. 2007 ). Although this tool was primarily designed to examine the quality of studies in the field of medical education, it is in practice subject neutral. As the MERSQI assesses not only the quality of experimental design and outcomes measures, but also the assessment instrumentation used, it was viewed as a suitable and comprehensive tool for quality appraisal. In addition, the same instrument was used in a previously peer-reviewed systematic review examining VR, by Jensen and Konradsen ( 2018 ).
The MERSQI tool covers six quality assessment domains. These include: study design, sampling, type of data, validity of evaluation instrument, data analysis, and outcomes. Each domain is scored out of three, with a maximum overall score of 18. Unlike Jensen and Konradsen ( 2018 ), the current review gave full points in the study design category for experimental trials with participant randomisation, as well as appropriate pre-intervention measures. This decision was made as true randomised control trials featuring random sampling is unrealistic in I-VR pedagogical research, as the participant sample can only be drawn from an educational establishment.
Quality of studies
The first domain examined for quality was the study design of the papers. There were 20 studies (69%) that featured an experimental design with stated random allocation of participants between control and experimental group. The review featured nine studies (31%) that were quasi-experimental in nature, meaning there was non-random allocation of participants into experimental groups.
Only one of the studies featured participants being studied at more than one institution, with most of the studies included ( N = 28) only sampling from a single establishment. All studies produced response rates of over 75%, which means they were given the highest score in that domain.
In terms of the type of data presented, all included studies featured an objective measure of learning outcomes such as test scores or completion times. No studies used self-assessment on the part of the participant to gauge learning outcomes.
The most pronounced weakness of the studies included in the review was the validity of the evaluation instrument used to assess learning outcomes. This domain pertained to the physical assessment instrumentation such as the quiz, test, or questionnaire that was given to the participant. Only six of the included studies (21%) reported the internal structure sufficiently through dimensionality, measurement invariance, or reliability using the criteria set down by Rios and Wells ( 2014 ). In addition, only 10 studies (34%) stated how the content was validated, with the majority ( N = 19) not reporting this information. Only three studies (Kozhevnikov et al. 2013 ; Makransky et al. 2017 ; Molina-Carmona et al. 2018 ) appropriately outlined both the internal structure and validity of evaluation content. The majority of studies ( N = 16) did not report either item.
Of the 29 studies in the current review, 26 scored full marks on the data analysis domain with both an appropriate and sufficiently complex analysis and reporting of the findings. Three studies scored lower than this due to reporting descriptive statistics only (Angulo and de Velasco 2013 ; Babu et al. 2018 ; Ray and Deb 2016 ).
Overall, the average quality score of a study in this systematic review was 12.7 with a range of 10.5–14.5 (SD = 1.0). This was 1.8 points higher than the review carried out by Jensen and Konradsen ( 2018 ), which could in part be due to differences in study design criteria which was previously outlined. A full summary of the MERSQI scores for each study can be found in Table 2 in the Appendix.
Subject areas and learning domains
Table 1 provides a summary of all 29 articles that were included in the review. Studies were first categorised by the population that was sampled. Most I-VR studies took place in a higher education establishment (college or university) using undergraduate or postgraduate students ( N = 25). A smaller number of studies used high school pupils ( N = 2), or adult education students ( N = 2) such as those in vocational or work-based programmes.
Each of the included studies were then examined for the topic and subject area they pertained to. This was based upon the nature of the VR experience, participant pool, and intervention. In total, six main subject areas were identified. This included: medicine ( N = 4), science (biology, chemistry, and physics) ( N = 13), social science (human geography) ( N = 1), computer science ( N = 2), engineering and architecture ( N = 7), and safety education ( N = 1). One of the included studies (Molina-Carmona et al. 2018 ) did not neatly fit into one of the pre-defined categories as it utilised I-VR to teach abstract spatial concept abilities to multimedia engineering students. It was therefore categorised as ‘other’. Figure 2 shows the percentage of papers included by subject area.
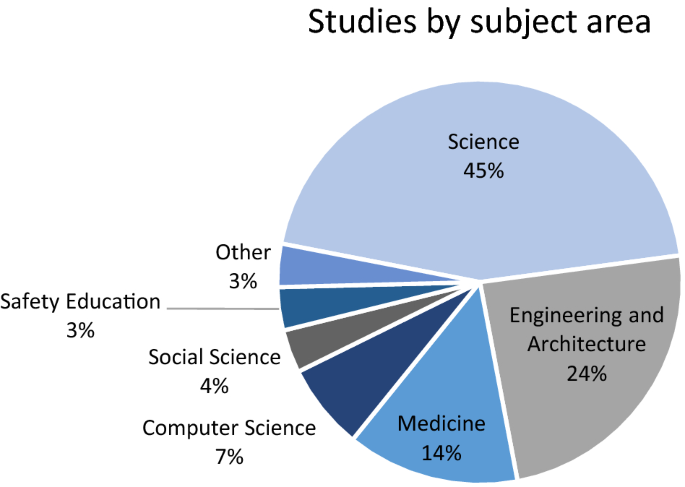
Percentage of papers per subject area
In addition to the subject area, the learning outcomes were also categorised into three specific domains based upon the findings of previous systematic reviews, as well as the taxonomy of learning developed by Bloom et al. ( 1956 ). The first was cognitive which related to studies that intended to teach specific declarative information or knowledge. The second was procedural which intends to teach the user how to perform a specific task or learn psychomotor skills that pertain to a certain activity. Finally, the third learning outcome was affective skills which can be defined as a growth in areas relating to emotion and attitude. Most of the included studies ( N = 24) concentrated on the cognitive domain, with two studies focusing on purely procedural and psychomotor skills. The remaining studies were a blend of two domains with Sankaranarayanan et al. ( 2018 ) and Smith et al. ( 2018 ) examining both cognitive and procedural skills, and Gutiérrez-Maldonado et al. ( 2015 ) utilising both cognitive knowledge and affective awareness in psychiatric diagnosis training. Figure 3 shows the percentage of studies included by learning domain.
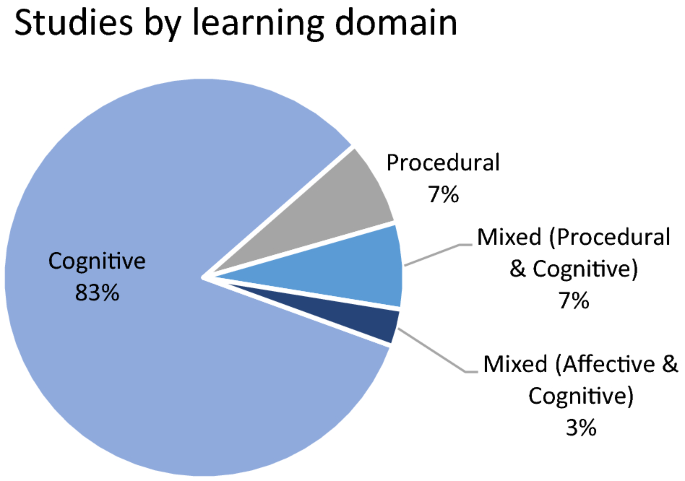
Percentage of papers per learning domain
Experimental design
Outcome measures.
A thorough understanding of the role of I-VR as a pedagogical practice can only be fully appreciated when consideration is given to the assessment instrumentation and outcome measures used to assess its utility. As previously mentioned, when analysing the quality of the included studies, it was the evaluation instrumentation itself that was shown to have the most pronounced weakness.
To assess the evaluation instruments being employed, the measures were broken down into two broad domains: outcome measures, and assessment instrumentation. Outcome measures can broadly be defined as how learning outcomes were quantified (e.g. by comparing test scores). Assessment instrumentation pertains to the evaluative instrument itself that is used to measure the learning outcomes (e.g. multiple-choice questionnaire, exam style questions). Twenty-seven of the included studies (93%) used test scores to assess learning outcomes, with the majority using this as their sole method. There were four studies that used completion time as a metric of learning outcome, although only one study (Bharathi and Tucker 2015 ) used this method exclusively. There was one study (Sankaranarayanan et al. 2018 ) that used the correct order of operation in a procedural task as one of its main outcome measures. There were three papers that utilised other outcome measures that could not be easily categorised. For instance Greenwald et al. ( 2018 ) used counting the number of moves needed to complete a task, Webster ( 2016 ) used the performance on a virtual jigsaw puzzle, and Angulo and de Velasco ( 2013 ) used a mixture of scores and evaluations of an architectural space.
Assessment instrumentation
In terms of the direct assessment instrumentation used to examine outcome measures, there was a heavy reliance on the multiple-choice questionnaire (MCQ). There were eighteen (62%) studies that utilised this method of assessment, with the majority of those using it as their sole evaluation instrument. Only five studies used extended answer questions (long or short form) to probe for a deeper understanding of the educational content, which was usually done in combination MCQs. The studies that included the teaching of procedural skills used marking criteria and checklists to assess whether the correct order was being followed. For instance Yoganathan et al. ( 2018 ) had an expert assessor use marking criteria to assess the knot tying skills of students. Similarly, Smith et al. ( 2018 ) had evaluators observe students with a decontamination checklist which evaluated performance based upon certain key tasks that were performed.
There were a smaller number of studies that used more novel instrumentation and methods for evaluation, such as the utilisation of labelling and identifying parts of a 3D model (e.g. Babu et al. 2018 ; Moro et al. 2017 ; Stepan et al. 2017 ). Fogarty et al. ( 2017 ) probed spatial and conceptual understanding in their assessment instrument by having participants draw shapes based on their understanding of structural engineering principles. Additionally, Alhalabi ( 2016 ) used quizzes on both mathematical knowledge, and the appropriate understanding of graphics and charts as an assessment measure for engineering students.
There were three studies (Liou and Chang 2018 ; Madden et al. 2018 ; Ray and Deb 2016 ) where the nature of the assessment instrumentation could not be definitively ascertained from the description.
The majority of studies (62%) utilised the pretest–posttest design by comparing the test scores pre-intervention with those after the I-VR experience. The remainder of the studies tended to assess post-intervention scores only, usually by comparing the difference in learning outcome between I-VR and one or more control group. Less conventional means of post-intervention comparison was sometimes utilised, such as Johnston et al. ( 2018 ) comparing the average score on a specific exam question that pertained to an I-VR experience that some student did or did not undertake.
There were four studies that examined the short to medium term retention rate of information and learning through follow-up assessment. This ranged from as soon as 1 day after the initial I-VR experience (Babu et al. 2018 ), through to 6 months post-intervention (Smith et al. 2018 ). Olmos-Raya et al. ( 2018 ) and Stepan et al. ( 2017 ) had follow-up assessments at 1-week and 8-weeks, respectively.
Intervention characteristics
In addition to having appropriate assessment measures, it is also important to examine the nature of the I-VR intervention itself. The most popular HMDs used were the Oculus ( N = 13) and HTC Vive ( N = 7). There were seven studies that used a form of mobile VR headset such as the Google Cardboard or Samsung Gear VR. In one study (Yoganathan et al. 2018 ) the exact HMD system used could not be definitively ascertained. Figure 4 provides a breakdown of the HMDs used in the included studies.
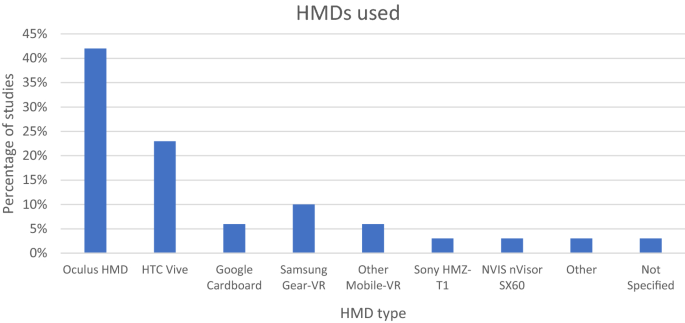
HMDs used in studies
Most studies (72%) featured only a single intervention with the I-VR experience, meaning that the student was exposed to the technology just once. There were a few exceptions to this, with Ostrander et al. ( 2018 ) having seven individual I-VR experiences in their manufacturing lesson, as well as Ray and Deb ( 2016 ) utilising smartphone based I-VR over the course of 16 sessions. Other studies allowed a greater degree of freedom in the number of interventions or times that a student could use I-VR. This was usually a result of time being dedicated to the technology through scheduled classes or lab times (e.g. Akbulut et al. 2018 ; Alhalabi 2016 ; Molina-Carmona et al. 2018 ). Despite this, the I-VR intervention was usually a single and isolated one.
As well as most of the studies featuring a single intervention, the exposure duration was also typically short, ranging from 6 to 30 mins. Generally, the exception to this was when the I-VR exposure lasted as long as it took the participant to complete a specific task, assessment, or procedure within the immersive environment (e.g. Babu et al. 2018 ; Bharathi and Tucker 2015 ; Greenwald et al. 2018 ; Sankaranarayanan et al. 2018 ). Molina-Carmona et al. ( 2018 ) supplemented the limited intervention duration by allowing participants to take the HMD away with them, so they could access the educational content for 2 weeks outside the classroom. However, just as with the number of interventions, exposure duration tended to be short, lasting on average 13 mins for those I-VR experiences that had a set time limit.
Most of the studies (62%) utilised I-VR as the sole method of learning, and did not combine the technology with additional pedagogical practices or materials to encourage learning. Only a limited number of studies (38%) supplemented the I-VR lesson by providing additional aids that were designed to complement the educational experience. For example, Smith et al. ( 2018 ) and Stepan et al. ( 2017 ) both had participants use web-based modules and textbooks in addition to the I-VR experience before testing them on learning outcomes. A number of the included studies also utilised lecture based instruction or scheduled class time to operate in tandem with the I-VR environments (e.g. Akbulut et al. 2018 ; Fogarty et al. 2017 ; Johnston et al. 2018 ; Ray and Deb 2016 ; Sankaranarayanan et al. 2018 ).
Theoretical frameworks
A fundamental component of any educational tool or activity is to ground its use in learning theory or educational paradigms. Learning theories can broadly be broken down and defined by proposals regarding how student imbibe, process, and retain the information that they have learned (Pritchard 2017 ; Schunk 2011 ). When applied to educational I-VR, these theories should provide a pedagogical framework and foundation as how best to design interventions. Papers were examined for explicit statements regarding the theoretical basis for the study. Those papers that only mentioned theoretical approaches as part of the introduction or literature review were not deemed to have explicitly stated them. The majority of studies ( N = 24) made no mention of a theoretical approach underpinning the intervention. There were two studies that applied a generative learning framework (Makransky et al. 2017 ; Parong and Mayer 2018 ). This can be defined as an approach where the learner will actively integrate new knowledge with information that is already stored in the brain (Osborne and Wittrock 1985 ). Webster ( 2016 ) employed Mayer's ( 2009 , 2014 ) Cognitive Theory of Multimedia Learning (CTML). CTML proposes a dual channel approach where visual and auditory information is actively processed, organised, and then stored in the brain. This is contingent on neither channel (visual or auditory) becoming overloaded with information. Smith et al. ( 2018 ) used the NLN Jeffries Simulation Theory as their theoretical basis. This theory, most commonly employed in nursing education, is where students learn information as part of a simulated experience (Jeffries et al. 2015 ). For the teaching of vocational skills, Babu et al. ( 2018 ) stated that their approach aligned with situated learning. Situated learning employs a constructivist approach in that students learns professional skills by actively participating in the experience (Huang et al. 2010 ).
Learning outcomes
For I-VR to gain wide-spread acceptance as a reliable pedagogical method, it must be shown to confer a tangible benefit in terms of learning outcomes over less immersive or traditional teaching methods.
Cognitive studies
There were twenty-four included studies that fell into the cognitive domain and aimed to teach specific declarative information or knowledge through the I-VR environment. The current review found that most studies demonstrated benefits in terms of learning outcomes when using I-VR compared to less immersive methods of learning. A smaller number of studies found no significant advantage regardless of the pedagogical method being utilised. The results of these cognitive studies have been broken down by subject area.
Science based cognitive studies
The review found that cognitive learning activities requiring a high degree of visualisation and experiential understanding may be best facilitated using immersive technologies. For instance, both Liou and Chang ( 2018 ) and Maresky et al. ( 2019 ) found that anatomical learning facilitated by complex 3D visualisations of the human body were more conducive to learning in I-VR compared to traditional learning or independent study. Similarly Lamb et al. ( 2018 ) used a virtual environment that allowed for the manipulation and movement of strands of DNA, which produced better learning outcomes in content tests than a lecture or a serious educational game. Greater attention and engagement with the I-VR environment as measured with infrared spectroscopy was one of the possible explanations given for the effectiveness of the technology. In a study by Johnston et al. ( 2018 ), participants volunteered to take part in a cell biology experience either because they were engaged with the subject matter itself, or wanted supplementary instruction. Johnston et al. ( 2018 ) compared the exam scores of those students who volunteered to take part with those who did not. The study found that participants who underwent the I-VR experience scored 5% higher on the related exam question compared to the rest of the assessment. Those who did not undergo the cell biology I-VR experienced scored on average 35% worse on the same question.
The increase in graphical fidelity afforded by I-VR has allowed not only for the creation of complex computer-generated environments, but also the viewing of high resolution 360° video. In one such study, Rupp et al. ( 2019 ) had participants watch a six minute 360° video about the International Space Station with either a HMD which created a sense of immersion and presence, or on a mobile screen. The research found that those participants in the HMD condition scored significantly higher in a learning outcome test (MCQ) than those who watched the video in the non-immersive condition.
Although I-VR has been shown to confer a benefit in science education, there is evidence to suggest that not all learning objectives can be learned equally well. For instance, in task devised by Allcoat and von Mühlenen ( 2018 ), the researchers found that I-VR conferred a benefit over video or textbook learning when questions required remembering , but not ones pertaining to understanding of the material. The authors suggest that unfamiliarity and the novelty of the I-VR environments could have contributed to the lack of an obvious benefit in the latter domain. Another study that examined specific question types to understand I-VR’s effectiveness was undertaken by Kozhevnikov et al. ( 2013 ). In this study, participants learned more conceptual and abstract relative motion concepts using either I-VR or D-VR. The study demonstrated that those in the I-VR condition performed significantly better in the two-dimensional problems than their D-VR counterparts, although there was no significant difference between groups in problems featuring only one spatial dimension.
There were several studies in the domain of science that showed no obvious benefits to using I-VR over traditional pedagogical methods. Two studies (Greenwald et al. 2018 ; Moro et al. 2017 ) compared science learning in I-VR with desktop based VR and 2D videos. Results showed no clear benefit of I-VR based instruction when comparing the difference and significance of learning outcomes between mediums. Similarly, Stepan et al. ( 2017 ) found that I-VR was no more effective than online textbooks for the teaching of neuroanatomy. Interestingly, the same study found no difference in information retention rates when the participants were reassessed 8-weeks later. Madden et al. ( 2018 ) used I-VR, D-VR, and the traditional ball and stick method to teach astronomy principles pertaining to phases of the moon. The study found that I-VR and D-VR produced comparable test score results, with no significant differences in attainment. However, the authors commented on the encouraging finding that despite being a novel technology to most participants, I-VR still facilitated comparable learning outcomes to more traditional methods.
Despite the majority of studies demonstrating that I-VR learning is more effective or at least on par with traditional pedagogical methods, some studies have shown a detrimental effect of I-VR. Makransky et al. ( 2017 ) used a combination of assessment and EEG to find that an I-VR lab simulation produced significantly poorer test scores than a non-immersive alternative. Similarly, during another science experiment, Parong and Mayer ( 2018 ) found that students who used I-VR during a biology lesson scored significantly poorer than those who learned using a PowerPoint. Both of these studies cited Mayer's ( 2009 , 2014 ) Cognitive Theory of Multimedia Learning as a possible explanation for the poorer performance for I-VR. The researchers postulate that the high-fidelity graphics and animations could have significantly increased cognitive load, which would have detracted from the learning task at hand. It was therefore proposed that a less immersive, yet well designed PowerPoint presentation would facilitate better learning outcomes than a graphically rich I-VR experience.
Engineering and architectural based cognitive studies
I-VR was effective in engineering and architectural education as a tool to visualise key concepts within the discipline. For example, Fogarty et al. ( 2017 ) allowed students to volunteer for an I-VR experience who struggled with the comprehension of spatial arrangements in structural engineering. Before the intervention, those students who volunteered to take part scored significantly poorer than their non-intervention counterparts. At post-test, not only did those who underwent the I-VR experience score significantly higher than they did at pre-test, but they also eliminated the significant difference with the non-intervention group. This would suggest that I-VR could serve an important function in supplementing or assisting learning in those students who are struggling to grasp complex problems relating to their discipline. Interestingly, Angulo and de Velasco ( 2013 ) used many of these same spatial and visualisation principles in a more applied setting. Their study split students into groups who were tasked with designing an architectural space (a health clinic waiting room), either with the assistance of an I-VR design tool (experimental group) or a physical model (control group). The study found the space that gained the most positive affect was designed by the I-VR group.
Webster ( 2016 ) created a graphically rich immersive environment which combined active and passive media with elements of gamification and interactivity to teach corrosion concepts to US army personnel. The study found that although both the I-VR environment and a traditional lecture were effective pedagogical methods for teaching these principles, it was the I-VR condition that produced the highest gain in knowledge acquisition.
There was also some evidence to suggest that I-VR interventions could assist in short-term retention of information in engineering related activities. Babu et al. ( 2018 ) found that although participants performed similarly in a mechanical labelling task using either I-VR or a tablet computer immediately post-intervention, the I-VR group had better retention of knowledge when the test was re-administered 1 day later. Furthermore, those participants in the I-VR group were also less likely to wrongly recall information compared to the non-immersive group on the retention test.
Interestingly, Ostrander et al. ( 2018 ) examined cognitive learning outcomes over seven separate manufacturing tasks utilising I-VR in one group, and a traditional class-based environment in the other. The study found that in six out of seven tasks, I-VR was no more effective than a traditional class where students could interact with the instructor or the physical models that they were accustomed to.
Medical based cognitive studies
Although papers featuring surgical simulators did not form part of this review, there were several applications of I-VR in the field of general medical education. Harrington et al. ( 2018 ) had medical students watch a ten-minute 360° video with slides containing surgical information superimposed over it. This was viewed either on a large television screen, or through a Gear VR headset. The study found no significant differences in knowledge retention scores between those who viewed the information through a HMD, or a traditional television screen. Despite not showing a distinct advantage in cognitive learning outcomes, the authors did suggest that the 360° surgical experience may facilitate a better understanding of how teamwork and interaction takes place within an operating theatre. This type of learning may be more difficult to measure using assessment instrumentation such as the MCQ, but nevertheless it could be that the experiential nature of I-VR may facilitate an understanding of interactions and communications. Smith et al. ( 2018 ) used either I-VR or D-VR on a computer to teach students about decontamination protocols. The research found that I-VR was no more effective than D-VR in a MCQ immediately post-intervention, or at 6-weeks follow-up.
Computer science based cognitive studies
Two studies demonstrated a significant advantage in using I-VR to teach computer science information. For instance, Akbulut et al. ( 2018 ) found that students who underwent an I-VR experience that focused on software engineering principles scored 12% higher than students who did not undergo I-VR learning. Interestingly, in a study by Ray and Deb ( 2016 ) that ran over 16 sessions on microcontrollers in computing, the I-VR group performance lagged behind that of the control group who used slideshows for the first four sessions. It was only on session number five that the I-VR group outperformed the control group, and this performance enhancement remained relatively stable in the majority of the remaining 11 sessions. In effect, it took the I-VR group some time to catch up with the control group, but once they did, they tended to outperform them in the remaining lessons. The authors propose that this may have been due to the novelty of the I-VR equipment which participants took time to become comfortable and competent with.
Other cognitive studies
I-VR was also used by Molina-Carmona et al. ( 2018 ) as a means of spatial ability acquisition and visualisation. The study showed that learning outcomes as assessed by a spatial visualisation test were higher among those who undertook the task in an immersive, compared to a non-immersive environment. There was only one study in the field of social science that used I-VR to teach cognitive information. Olmos-Raya et al. ( 2018 ) used either I-VR or a tablet-based system to teach high school students about human geography. The research found that I-VR produced higher learning gains on a MCQ than the tablet-based system. Further, those who used I-VR performed better than the non-immersive group on a knowledge retention quiz when administered 1-week later.
Procedural studies
Three of the four studies that attempted to utilise I-VR as a means of teaching procedural skills showed a distinct advantage over less immersive methods. Bharathi and Tucker ( 2015 ) found that engineering students were faster in assembling a household appliance in a virtual functional analysis activity in I-VR compared to D-VR. Yoganathan et al. ( 2018 ) also found that medical students were more accurate in knot tying practice when using I-VR as a training tool as opposed to a control group who used a standard video. Medical and surgical residents were also studied by Sankaranarayanan et al. ( 2018 ) who used I-VR as a teaching tool for emergency fire response in an operating theatre environment. This study found that 70% of those who utilised the I-VR training were able to perform the correct procedure in the correct order. This was 50% higher than the control group who were exposed to a presentation and reading material only and did not experience I-VR.
One of the studies found no significant advantage to using I-VR as a learning tool. Smith et al. ( 2018 ) split nursing students into an I-VR group, a D-VR group (desktop PC based), or a written instruction group to learn about appropriate protocols for decontamination. The study found that there was no significant difference in performance between the groups as measured by a decontamination checklist, or the time taken to complete the task. Furthermore, reassessment 6 months later showed that I-VR conferred no advantage in procedural knowledge retention (accuracy and speed) compared to less immersive methods.
Affective studies
Only one of the studies attempted to use I-VR as a pedagogical tool to teach applied behavioural and affective skills. Gutiérrez-Maldonado et al. ( 2015 ) used I-VR in the field of diagnostic psychiatry in an attempt to improve interview skills when assessing patients for an eating disorder. Participants were exposed to a series of virtual patient avatars in either the I-VR condition, or a D-VR condition using stereoscopic glasses. Analysis showed that both conditions were equally as effective, and no significant differences were shown in the acquisition of skills between the two groups. Nevertheless, this was a novel study as it traversed the boundaries between traditional cognitive skill acquisition and applied behavioural and affective change.
Discussion and implications
The purpose of this review was to investigate I-VR’s effectiveness as a pedagogical method in education, as well as examining the experimental design and characteristics of the included studies. In particular, the review found that the utilisation of I-VR is typically restricted to a small number of subject areas such as science and engineering. Furthermore, a heavy reliance has been placed on the MCQ and test score measures to assess learning outcomes. In addition, I-VR interventions were typically short and isolated, and were not complemented with additional or supplementary learning material. Despite this, most studies did find a significant advantage of using I-VR over less immersive methods of learning. This was the case particularly when the subject area was highly abstract or conceptual, or focused on procedural skills or tasks.
Is the utilisation of I-VR within education restrictive?
The findings of the review suggest a relatively homogenous application of I-VR in terms of both the subject areas represented, as well as the learning domain being taught. Almost 70% of the studies were from the field of science or engineering, with other subjects being marginally represented. It is worth noting, however, that although medical disciplines made up a small proportion of the studies included (14%), this was because most medical applications of I-VR feature surgical simulators and therefore were not part of the current review’s inclusion criteria. Most studies utilised I-VR as a way of teaching cognitive skills, with only a handful examining the procedural or affective applications.
The findings of the review raise several issues when trying to assess the general effectiveness of I-VR in education. Similar to the findings of others (e.g. Jensen and Konradsen 2018 ; Radianti et al. 2020 ), the arts, humanities, and social sciences were underrepresented in in the current review. This makes generalisable conclusions as to the cognitive benefit of the technology in these subjects challenging. One major reason for this under representation may be the lack of I-VR learning content, experiences, and teaching tools. Jensen and Konradsen ( 2018 ) highlighted that instructors are restricted to the material published and produced by VR designers, and this may not necessarily meet the individual needs of the teacher, or the learning outcome trying to be achieved. The skillset needed to produce and create wholly virtual environments that can be rendered and displayed in a HMD is still demanding, despite the release of affordable VR creation suites. Therefore, the bespoke I-VR experiences required to teach social science lessons (or indeed any subject) is completely dependent on an appropriate I-VR tool already existing or having the technical proficiency to create one. A potential solution to the lack of bespoke material could be the examination of the pedagogical effectiveness of HMD 360° video in the classroom, as opposed to computer-generated environments. This content is comparatively easier to create using appropriate video equipment and can be tailored to the individual needs of the instructor or student group. Widespread research that examines the potential of I-VR in a multitude of diverse disciplines and learning domains will continue to be constrained by the availability of the requisite material. That is until such a time where bespoke and individually tailored I-VR experiences become more accessible.
Implications of outcome measures and assessment instrumentation
One of the most striking characteristics of the assessment instrumentation used in the studies was the reliance on the MCQ to assess learning outcomes. Although there have been many debates on the respective advantages and disadvantages of utilising the MCQ, it has generally been considered that it is most appropriate for testing large amounts of surface knowledge over the course of an entire module or syllabus (Excell 2000 ). As O’Dwyer ( 2012 ) points out, the assessment instrumentation encourages comprehensive learning of the entirety of the taught material, as opposed to just specific components. However, since most of the studies featured single interventions of between 6 and 30 mins, doubts are cast on whether MCQs are the most appropriate way to assess learning. Since the MCQ was most commonly administered immediately after the I-VR experience, much of the information may still be stored in short-term memory, and this may not give an accurate reflection of more comprehensive learning or long-term retention.
A second disadvantage associated with the heavy reliance on the MCQ is the limited breadth of knowledge that can be assessed. In Jensen and Konradsen’s ( 2018 ) systematic review, the researchers found that none of the cognitive studies went beyond teaching lower level cognitive skills as defined by Bloom’s taxonomy (Bloom et al. 1956 ). Similar results were found in the current review, with most studies requiring only a knowledge of previously learned material to successfully achieve the desired learning goal. Previous research on pedagogical assessment material (e.g. Ozuru et al. 2013 ) has suggested that the MCQ cannot assess higher levels of cognitive understanding or conceptual knowledge. Therefore, it may not only be the nature of the I-VR experience itself that restricts the learning of higher level cognitive skills, but also the restrictive nature of the assessment instrumentation that may impede an appropriate demonstration of learning outcomes. The utilisation of short or long form answers could be able to provide a more appropriate measure of the depth of learning achieved, giving the student an opportunity to demonstrate their conceptual knowledge of a given subject. Furthermore, I-VR research could benefit by expanding the very definition of what constitutes a learning outcome. This could be achieved by not relying exclusively on test score comparisons, but rather examine how I-VR could be used to foster deeper conceptual understanding through experiential learning and subsequent classroom discussions with peers or instructors.
Implication of intervention characteristics for learning outcomes
The current review examined how I-VR is being utilised in experimental and applied settings, and the implications this has for assessing its pedagogical suitability. In most studies, the participant took part in a single I-VR experience that was also short in duration. This presents several key challenges. Most importantly, the novelty of the I-VR technology itself may have impeded the learning experience of the user, especially if they had never used the technology before or were unfamiliar with it. This seemed to be demonstrated by Ray and Deb ( 2016 ) who found that in the initial sessions of I-VR learning, performance was on average poorer than those who underwent traditional teaching methods. It was only after the participants began to become familiar with the technology (on session number five) that learning surpassed the control group. Similarly, studies that allowed for extended exposure to I-VR (e.g. Akbulut et al. 2018 ; Alhalabi 2016 ; Molina-Carmona et al. 2018 ), either through free navigation, repeated sessions, or scheduled class time, tended to show an advantage of using I-VR over non-immersive or traditional methods. It is therefore important to address the potentially negative influence that I-VR’s novelty as a learning tool may have, especially when outcomes are directly compared to another medium or method. Scepticism for media comparison studies was highlighted in the 1980s by Clark ( 1983 ), and then later re-addressed by Parong and Mayer ( 2018 ). As Parong and Mayer ( 2018 ) put it, the side-by-side comparison of two learning methods is an “apples-to-oranges type of comparison” (p. 788). This “apples-to-oranges” comparison is made starker when considering that I-VR is an unfamiliar technology to most in an educational capacity, and its pedagogical outcomes are being directly compared with familiar methods such as textbooks or lectures. It is important to consider that the novelty of HMDs and I-VR may hinder learning outcomes and classroom application, and it is therefore prudent to ensure that the degree of familiarity with I-VR technology is factored into any direct comparison with other methods. In practice, this could mean that participants require extended familiarisation trials or free navigation before the start of experimental studies as a means of mitigating against potential problems caused by technological novelty.
In addition to the short intervention and exposure time, most studies did not complement I-VR with an additional method of teaching or self-learning. The limited number of studies that did tended to utilise web-based textbooks or modules, as well as lectures and scheduled class time. Encouragingly, those studies that combined or supplemented traditional class-based learning with I-VR (e.g. Akbulut et al. 2018 ; Fogarty et al. 2017 ; Johnston et al. 2018 ; Sankaranarayanan et al. 2018 ; Yoganathan et al. 2018 ) tended to show a learning advantage. This suggests that I-VR may be best employed as form of blended or multi-modal learning to supplement and complement class-based instruction (Garrison and Kanuka 2004 ). An area for investigation would be to examine I-VR’s application longitudinally in a natural classroom environment. The current review contained only a limited number of studies that employed this approach, however, by implementing and studying how I-VR can be adopted and integrated into a module or syllabus, a clearer picture of its capabilities can emerge.
Learning theories ultimately provide a theoretical framework and foundation as how best to design educational interventions (Pritchard 2017 ; Schunk 2011 ). However, the review found that few papers explicitly state that any predetermined learning theory was used to advise the characteristics or methods of the study. Similar findings were reported in a systematic review by Radianti et al. ( 2020 ) examining I-VR use in higher education exclusively. Radianti et al.’s ( 2020 ) review found that in around 70% of the 38 studies included, no learning theory was mentioned as forming the foundation of the VR activity. Several studies have shown that educators regard clear pre-defined intervention characteristics and objectives as essential components of I-VR teaching (Fransson et al. 2020 ; Lee and Shea 2020 ). It is therefore essential that future experimental and applied research is based on a sound theoretical basis that can advise how the technology can be appropriately utilised and assessed.
Learning outcomes in I-VR
The current review examined learning outcomes across three domains: cognitive, procedural, and affective. By far the most popular domain was the teaching of cognitive skills and knowledge which made up 83% of the studies in the current review. Around half of those demonstrated a positive effect on learning when using I-VR over less immersive pedagogical methods. Most of the remaining studies showed no significant effect either way, with only a small number of papers exhibiting detrimental results. Researchers have suggested that the increased levels of immersive content that stimulate multisensory engagement can ultimately lead to more effective learning outcomes (Webster 2016 ). When this is implemented in cognitive learning activities that require a high degree of spatial understanding and visualisation (e.g. Maresky et al. 2019 ), I-VR can allow the user to gain insights that are difficult to reproduce in reality. This review has already identified scientific subjects such as biology and physics as promising avenues for educational I-VR implementation. However, other scientific disciplines that require abstract or conceptual understanding (e.g. chemistry, mathematics) could also benefit from the visualisation afforded by I-VR.
Studies that utilised I-VR for the teaching of procedural skills and knowledge produced encouraging results, with three of the four studies finding a significantly positive increase in learning (Bharathi and Tucker 2015 ; Sankaranarayanan et al. 2018 ; Yoganathan et al. 2018 ). Interestingly, two of the studies featured a transfer component by having the user first practice the procedure in I-VR, and then use this form of experiential learning to complete a task in the real world. Yoganathan et al. ( 2018 ) had students practice how to tie a surgical knot in I-VR and then complete this task for real in-front of an expert. Sankaranarayanan et al. ( 2018 ) had medical students learn how to deal with an operating theatre fire by first practicing the procedure in I-VR, and then applying this knowledge to a mock emergency in a real operating room. Both studies found a positive effect of using I-VR as the training method by demonstrating improved results when performed in a real environment. These are encouraging findings for I-VR’s effectiveness in psychomotor and procedural education, as there has been a degree of scepticism over whether I-VR simply produces a “getting good at the game” effect. For instance, Jensen and Konradsen ( 2018 ) point out that the honing of procedural skills within I-VR may simply lead to the participant becoming proficient when performing the task virtually, and this may not necessarily transfer to the real world. The current review has identified that the two procedural studies that implemented a transfer task did indeed demonstrate a significant benefit to using I-VR as an initial education method. This demonstrates that virtual training can be a successful precursor to implementation in the real world. This suggests that I-VR could be useful in educating students in dangerous vocational subjects such as electrical engineering without risk to themselves or others. However, this view is based on a small number of studies, and it is therefore important that future procedural tasks utilise a transfer activity to understand the potential scope and parameters surrounding I-VR training and real-world application.
Only one of the studies had a firm focus on the training of affective skills, namely by using I-VR as a way of teaching diagnostic interview techniques in a psychiatric setting (Gutiérrez-Maldonado et al. 2015 ). Although this study found no clear advantage to using I-VR, other research out with the domain of education has demonstrated promising results in utilising the technology for affective and behavioural change. This included applying the technology successfully in areas such as exposure therapy, anxiety disorder treatment, and empathy elicitation (Botella et al. 2017 ; Maples-Keller et al. 2017a , b ; Schutte and Stilinović 2017 ). As a result of the strong non-educational body of literature suggesting I-VR can facilitate affective and behavioural change, future research should examine how this can be applied in an educational context, and then transferred to real-world scenarios. For instance, in their psychiatric interview experience, Gutiérrez-Maldonado et al. ( 2015 ) had users interact solely with virtual avatars, and did not have the participants demonstrate their learning with a real actor or patient. Therefore, just like with procedural skill acquisition, affective I-VR experiences should seek to understand how virtual learning can then be applied to real situations.
Implications and future practice
The current review has been able to identify a body of experimental and applied research that show the potential benefits of using I-VR in education. It has already been noted that I-VR has traditionally been used to teach low level or fundamental skills and knowledge, and has not necessarily been used to facilitate what Bloom et al. ( 1956 ) would consider higher level learning. This would include analysing and evaluating experience. By expanding the definition of learning outcomes to encompass potential benefits such as an increased depth of understanding or the ability to identify complex themes, pedagogical practice can take advantage of the inherent strength of the medium. These should be comprehensively analysed to investigate learning outcomes that go beyond simple test scores.
The review has also been able to identify areas for improvement in future studies, which would address confounding variables and expand the scope of research. Firstly, as Allcoat and von Mühlenen ( 2018 ) suggest, the novelty of I-VR could hamper learning outcomes due to unfamiliarity with the technology. Therefore, it is important to factor in an extended familiarisation or free navigation period that would help alleviate this concern. Additionally, follow-up qualitative analysis such as interviews or focus groups could help explore the phenomenology or direct experience of using I-VR, and highlight concerns relating to unfamiliarity or technological anxiety. The biggest concern relating to the assessment instrumentation was the over reliance on the MCQ (62% of studies used it as the sold method of assessment). Although this method is deemed appropriate for assessing large amounts of surface knowledge, it may not reveal more nuanced forms of learning that extend beyond mere recall of information. Therefore, long form essay questions, oral examinations, or group discussions could be used to facilitate students’ ability to present their in-depth understanding and applied knowledge. Future research must base the nature of these interventions on a sound theoretical framework. This would assist in identifying specific learning objectives and methods of assessments. An explicit theoretical approach was commonly lacking in the included studies.
I-VR has already been demonstrated to be an effective tool in non-pedagogical behaviour change, such as treating phobias, mental health conditions, or as a tool for rehabilitation (Botella et al. 2017 ; Maples-Keller et al. 2018; Ravi et al. 2017 ). Research should therefore concentrate on I-VR’s potential as an acquisition tool for affective skills. There is already a strong body of evidence suggesting I-VR experiences can elicit high levels of empathetic response and perspective taking, and this should be explored within an educational context (Herrera et al. 2018 ; Shin 2018 ). For example, Dyer et al. ( 2018 ) used I-VR to allow health care students to take the perspective of an older patient with age-related medical conditions, which led to increased empathy. Future studies should investigate whether this perspective taking ability can lead to higher domains of learning, such as evaluating one’s actions, applying problem solving skills, or creating new solutions as a direct result of the insights they received from I-VR. This will require researchers and instructors to carefully consider their tools for evaluation and assessment, perhaps incorporating mixed-methods to give a more holistic overview of learning achieved.
Conclusions
The current review found that I-VR conferred a learning benefit in around half of cognitive studies, especially where highly complex or conceptual problems required spatial understanding and visualisation. Although many studies found no significant benefit of using I-VR over less immersive technology, only a small number resulted in detrimental effects on learning outcomes. However, the homogenous nature of assessment instrumentation, such as an over reliance on the MCQ may have stifled the ability for participants to demonstrate learning outcomes beyond low level cognitive knowledge. Short exposure times and isolated interventions could also pose a problem as the novel nature of the technology could negatively impact the amount of learning able to be imbibed. Encouragingly, most procedural tasks did show a benefit to utilising I-VR, and furthermore, there was evidence that virtual skill acquisition could be transferred successfully to real world problems and scenarios. The ability to repeatedly practice a procedure in a safe environment whilst expending little resources could be one of the most advantageous and intrinsic benefits of I-VR technology. Although affective behavioural change has been widely studied in non-educational applications of I-VR, the domain was underrepresented in the current review, and is an important area for future investigation.
Over the coming years, technological advancement, an increase in creative content, and the possibilities for instructors to create bespoke I-VR experiences will all contribute to I-VR’s potential as a teaching tool. It is essential therefore that the implementation of such technology is based on sound theoretical and experimental evidence in order to ensure that the I-VR is utilised correctly, and to its full potential.
Akbulut, A., Catal, C., & Yıldız, B. (2018). On the effectiveness of virtual reality in the education of software engineering. Computer Applications in Engineering Education, 26 , 918–927.
Google Scholar
Alaraj, A., Lemole, M., Finkle, J., Yudkowsky, R., Wallace, A., Luciano, C., … Charbel, F. (2011). Virtual reality training in neurosurgery: Review of current status and future applications. Surgical Neurology International , 2 , 52
Alhalabi, W. S. (2016). Virtual reality systems enhance students’ achievements in engineering education. Behaviour & Information Technology, 35 , 919–925.
Allcoat, D., & von Mühlenen, A. (2018). Learning in virtual reality: Effects on performance, emotion and engagement. Research in Learning Technology, 26 , 2140.
Angulo, A., & de Velasco, G. V. (2013). Immersive simulation of architectural spatial experiences. Blucher Design Proceedings, 1 , 495–499.
Babu, S., Krishna, S., Unnikrishnan, R., & Bhavani, R. (2018). Virtual reality learning environments for vocational education: A comparison study with conventional instructional media on knowledge retention. In 2018 IEEE 18th International Conference on Advanced Learning Technologies (ICALT) (pp. 385–389). IEEE.
Bailenson, J. N., Markowitz, D. M., Pea, R. D., Perone, B. P., & Laha, R. (2018). Immersive virtual reality field trips facilitate learning about climate change. Frontiers in Psychology, 9 , 2364.
Bharathi, A. K. B. G., & Tucker, C. S. (2015). Investigating the impact of interactive immersive virtual reality environments in enhancing task performance in online engineering design activities. In ASME 2015 International Design Engineering Technical Conferences and Computers and Information in Engineering Conference . ASME.
Bloom, B. S., Englehard, M. D., Furst, E. J., Hill, W. H., & Krathwohl, D. (1956). Taxonomy of educational objectives, handbook I: The cognitive domain . New York, NY: Longmans.
Botella, C., Fernández-Álvarez, J., Guillén, V., García-Palacios, A., & Baños, R. (2017). Recent progress in virtual reality exposure therapy for phobias: A systematic review. Current Psychiatry Reports, 19 , 42.
Brown, A., & Green, T. (2016). Virtual reality: Low-cost tools and resources for the classroom. TechTrends, 60 , 517–519.
Çalişkan, O. (2011). Virtual field trips in education of earth and environmental sciences. Procedia-Social and Behavioral Sciences, 15 , 3239–3243.
Clark, R. E. (1983). Reconsidering research on learning from media. Review of Educational Research, 53 , 445–459.
Dickey, M. D. (2005). Three-dimensional virtual worlds and distance learning: Two case studies of Active Worlds as a medium for distance education. British Journal of Educational Technology, 36 , 439–451.
Dyer, E., Swartzlander, B. J., & Gugliucci, M. R. (2018). Using virtual reality in medical education to teach empathy. Journal of the Medical Library Association, 106 , 498–500.
Ellinger, R. S., & Frankland, P. (1976). Computer-assisted and lecture instruction: A comparative experiment. Journal of Geography, 75 , 109–120.
Excell, P. S. (2000). Experiments in the use of multiple-choice examinations for electromagnetics-related topics. IEEE Transactions on Education, 43 , 250–256.
Fogarty, J., McCormick, J., & El-Tawil, S. (2017). Improving student understanding of complex spatial arrangements with virtual reality. Journal of Professional Issues in Engineering Education and Practice, 144 , 04017013.
Fransson, G., Holmberg, J., & Westelius, C. (2020). The challenges of using head mounted virtual reality in K-12 schools from a teacher perspective. Education and Information Technologies., 2 , 20–22.
Freina, L., & Ott, M. (2015). A literature review on immersive virtual reality in education: State of the art and perspectives. In The 11th International Scientific Conference ELearning and Software for Education (pp. 133–141).
Garrison, D. R., & Kanuka, H. (2004). Blended learning: Uncovering its transformative potential in higher education. Internet and Higher Education, 7 , 95–105.
Gee, J. P. (2004). What video games have to teach us about learning and literacy. Education Training, 46 , 20.
Greenwald, S. W., Corning, W., Funk, M., & Maes, P. (2018). Comparing learning in virtual reality with learning on a 2D screen using electrostatics activities. Journal of Universal Computer Science, 24 , 220–245.
Gutiérrez-Maldonado, J., Ferrer-García, M., Pla-Sanjuanelo, J., Andrés-Pueyo, A., & Talarn-Caparrós, A. (2015). Virtual reality to train diagnostic skills in eating disorders. Comparison of two low cost systems. Studies in Health Technology and Informatics, 219 , 75–81.
Häfner, P., Dücker, J., Schlatt, C., & Ovtcharova, J. (2018). Decision support method for using virtual reality in education based on a cost-benefit-analysis. In 4th International Conference of the Virtual and Augmented Reality in Education, VARE 2018 , 103–112.
Harrington, C. M., Kavanagh, D. O., Wright Ballester, G., Wright Ballester, A., Dicker, P., Traynor, O., … Tierney, S. (2018). 360° operative videos: A randomised cross-over study evaluating attentiveness and information retention. Journal of Surgical Education , 75 , 993–1000
Herrera, F., Bailenson, J., Weisz, E., Ogle, E., & Zak, J. (2018). Building long-term empathy: A large-scale comparison of traditional and virtual reality perspective-taking. PLoS ONE, 13 , e0204494.
Hodgson, E., Bachmann, E. R., Vincent, D., Zmuda, M., Waller, D., & Calusdian, J. (2015). WeaVR: A self-contained and wearable immersive virtual environment simulation system. Behavior Research Methods., 47 (1), 296–307.
Hodgson, P., Lee, V. W. Y., Chan, J. C. S., Fong, A., Tang, C. S. Y., Chan, L., et al. (2019). Immersive virtual reality (IVR) in higher education: Development and implementation. In M. Dieck & T. Jung (Eds.), Augmented reality and virtual reality (pp. 161–173). New York: Springer.
Howard-Jones, P., Ott, M., van Leeuwen, T., & De Smedt, B. (2015). The potential relevance of cognitive neuroscience for the development and use of technology-enhanced learning. Learning, Media and Technology, 40 , 131–151.
Huang, H. M., Rauch, U., & Liaw, S. S. (2010). Investigating learners’ attitudes toward virtual reality learning environments: Based on a constructivist approach. Computers and Education, 55 , 1171–1182.
Jeffries, P. R., Rodgers, B., & Adamson, K. (2015). NLN Jeffries simulation theory: Brief narrative description. Nursing Education Perspectives, 36 , 292–293.
Jensen, L., & Konradsen, F. (2018). A review of the use of virtual reality head-mounted displays in education and training. Education and Information Technologies, 23 , 1515–1529.
Johnston, A. P. R., Rae, J., Ariotti, N., Bailey, B., Lilja, A., Webb, R. I., … Parton, R. G. (2018). Journey to the centre of the cell: Virtual reality immersion into scientific data. Traffic , 19 , 105–110
Kozhevnikov, M., Gurlitt, J., & Kozhevnikov, M. (2013). Learning relative motion concepts in immersive and non-immersive virtual environments. Journal of Science Education and Technology, 22 , 952–962.
Lamb, R., Antonenko, P., Etopio, E., & Seccia, A. (2018). Comparison of virtual reality and hands on activities in science education via functional near infrared spectroscopy. Computers and Education, 124 , 14–26.
Larsen, C. R., Oestergaard, J., Ottesen, B. S., & Soerensen, J. L. (2012). The efficacy of virtual reality simulation training in laparoscopy: A systematic review of randomized trials. Acta Obstetricia et Gynecologica Scandinavica, 91 , 1015–1028.
Lee, C., & Shea, M. (2020). Exploring the use of virtual reality by pre-service elementary teachers for teaching science in the elementary classroom. Journal of Research on Technology in Education, 52 , 163–177.
Lee, E. A. L., Wong, K. W., & Fung, C. C. (2010). How does desktop virtual reality enhance learning outcomes? A structural equation modeling approach. Computers & Education, 55 , 1424–1442.
Lee, J. (1999). Effectiveness of computer-based instructional simulation: A meta analysis. International Journal of Instructional Media, 26 , 71–86.
Li, L., Yu, F., Shi, D., Shi, J., Tian, Z., Yang, J., … Jiang, Q. (2017). Application of virtual reality technology in clinical medicine. American Journal of Translational Research , 9 , 3867–3880
Liou, W., & Chang, C. (2018). Virtual reality classroom applied to science education. In 2018 23rd International Scientific-Professional Conference on Information Technology (IT) (pp. 1–4).
Lyne, D. S. (2013). Development of virtual reality applications for the construction industry using the Oculus Rift head mounted display. In Proceedings of the 13th International Conference on Construction Applications of Virtual Reality (pp. 30–31).
Madden, J. H., Won, A. S., Schuldt, J. P., Kim, B., Pandita, S., Sun, Y., … Holmes, N. G. (2018). Virtual reality as a teaching tool for moon phases and beyond. In 2018 Physics Education Research Conference .
Makransky, G., Terkildsen, T. S., & Mayer, R. E. (2017). Adding immersive virtual reality to a science lab simulation causes more presence but less learning. Learning and Instruction, 60 , 225–236.
Maples-Keller, J. L., Bunnell, B. E., Kim, S.-J., & Barbara, R. (2017a). The use of virtual reality technology in the treatment of anxiety and other psychiatric disorders. Harvard Review of Psychiatry, 25 , 103–113.
Maples-Keller, J. L., Yasinski, C., Manjin, N., & Rothbaum, B. O. (2017b). Virtual reality-enhanced extinction of phobias and post-traumatic stress. Neurotherapeutics, 14 , 554–563.
Maresky, H. S., Oikonomou, A., Ali, I., Ditkofsky, N., Pakkal, M., & Ballyk, B. (2019). Virtual reality and cardiac anatomy: Exploring immersive three-dimensional cardiac imaging, a pilot study in undergraduate medical anatomy education. Clinical Anatomy, 32 , 238–243.
Mayer, R. E. (2009). Multimedia learning (2nd ed.). Cambridge: Cambridge University Press.
Mayer, R. E. (2014). Cognitive theory of multimedia learning. In R. E. Mayer (Ed.), The Cambridge handbook of multimedia learning (2nd ed.). Cambridge: Cambridge University Press.
Merchant, Z., Goetz, E. T., Cifuentes, L., Keeney-Kennicutt, W., & Davis, T. J. (2014). Effectiveness of virtual reality-based instruction on students’ learning outcomes in K-12 and higher education: A meta-analysis. Computers and Education, 70 , 29–40.
Molina-Carmona, R., Pertegal-Felices, M., Jimeno-Morenilla, A., & Mora-Mora, H. (2018). Virtual reality learning activities for multimedia students to enhance spatial ability. Sustainability, 10 , 1074.
Moro, C., Štromberga, Z., Raikos, A., & Stirling, A. (2017). The effectiveness of virtual and augmented reality in health sciences and medical anatomy. Anatomical Sciences Education, 10 , 549–559.
Murcia-López, M., & Steed, A. (2016). The effect of environmental features, self-avatar, and immersion on object location memory in virtual environments. Frontiers in ICT, 3 , 1–10.
O’Dwyer, A. (2012). A teaching practice review of the use of multiple-choice questions for formative and summative assessment of student work on advanced undergraduate and postgraduate modules in engineering. The All Ireland Journal of Teaching and Learning in Higher Education, 4 , 1–12.
Olmos, E., Cavalcanti, J. F., Soler, J.-L., Contero, M., & Alcañiz, M. (2018). Mobile virtual reality: A promising technology to change the way we learn and teach. In S. M. Bell (Ed.), Mobile and ubiquitous learning: An international handbook (pp. 95–106). Singapore: Springer.
Olmos-Raya, E., Ferreira-Cavalcanti, J., Contero, M., Castellanos, M. C., Giglioli, I. A. C., & Alcañiz, M. (2018). Mobile virtual reality as an educational platform: A pilot study on the impact of immersion and positive emotion induction in the learning process. Eurasia Journal of Mathematics, Science and Technology Education, 14 , 2045–2057.
Osborne, R., & Wittrock, M. (1985). The generative learning model and its implications for science education. Studies in Science Education., 12 , 59–87.
Ostrander, J. K., Tucker, C. S., Simpson, T. W., & Meisel, N. A. (2018). Evaluating the effectiveness of virtual reality as an interactive educational resource for additive manufacturing. In Volume 3: 20th International Conference on Advanced Vehicle Technologies; 15th International Conference on Design Education , V003T04A018. ASME.
Ozuru, Y., Briner, S., Kurby, C. A., & McNamara, D. S. (2013). Comparing comprehension measured by multiple-choice and open-ended questions. Canadian Journal of Experimental Psychology, 67 , 215–227.
Parong, J., & Mayer, R. E. (2018). Learning science in immersive virtual reality. Journal of Educational Psychology, 110 , 785–797.
Pritchard, A. (2017). Ways of learning: Learning theories for the classroom (4th ed.). London: Routledge.
Radianti, J., Majchrzak, T. A., Fromm, J., & Wohlgenannt, I. (2020). A systematic review of immersive virtual reality applications for higher education: Design elements, lessons learned, and research agenda. Computers and Education, 147 , 103778.
Ravi, D. K., Kumar, N., & Singhi, P. (2017). Effectiveness of virtual reality rehabilitation for children and adolescents with cerebral palsy: An updated evidence-based systematic review. Physiotherapy, 103 , 245–258.
Ray, A. B., & Deb, S. (2016). Smartphone based virtual reality systems in classroom teaching - A study on the effects of learning outcome. In 2016 IEEE Eighth International Conference on Technology for Education (T4E) (pp. 68–71).
Reed, D. A., Cook, D. A., Beckman, T. J., Levine, R. B., Kern, D. E., & Wright, S. M. (2007). Association between funding and quality of published medical education research. JAMA, 298 , 1002.
Rios, J., & Wells, C. (2014). Validity evidence based on internal structure. Psicothema, 26 , 108–116.
Rupp, M. A., Odette, K. L., Kozachuk, J., Michaelis, J. R., Smither, J. A., & McConnell, D. S. (2019). Investigating learning outcomes and subjective experiences in 360-degree videos. Computers & Education, 128 , 256–268.
Sankaranarayanan, G., Wooley, L., Hogg, D., Dorozhkin, D., Olasky, J., Chauhan, S., … Jones, D. B. (2018). Immersive virtual reality-based training improves response in a simulated operating room fire scenario. Surgical Endoscopy and Other Interventional Techniques , 32 , 3439–3449
Schunk, D. H. (2011). Learning theories: An educational perspective (6th ed.). London: Pearson.
Schutte, N. S., & Stilinović, E. J. (2017). Facilitating empathy through virtual reality. Motivation and Emotion, 41 , 708–712.
Sherman, W. R., & Craig, A. B. (2003). Understanding virtual reality: Interface, application, and design. In Understanding Virtual Reality: Interface, Application, and Design .
Shin, D. (2018). Empathy and embodied experience in virtual environment: To what extent can virtual reality stimulate empathy and embodied experience? Computers in Human Behavior., 78 , 64–73.
Slater, M. (2018). Immersion and the illusion of presence in virtual reality. British Journal of Psychology, 109 , 431–433.
Smith, S. J., Farra, S. L., Ulrich, D. L., Hodgson, E., Nicely, S., & Mickle, A. (2018). Effectiveness of two varying levels of virtual reality simulation. Nursing Education Perspectives, 39 , 10–15.
Stepan, K., Zeiger, J., Hanchuk, S., Del Signore, A., Shrivastava, R., Govindaraj, S., et al. (2017). Immersive virtual reality as a teaching tool for neuroanatomy. International Forum of Allergy and Rhinology, 7 , 1006–1013.
UKAuthority. (2019). VR and AR attract education sector interest. Retrieved December 16, 2019, from https://www.ukauthority.com/articles/vr-and-ar-attract-education-sector-interest/
Webster, R. (2016). Declarative knowledge acquisition in immersive virtual learning environments. Interactive Learning Environments, 24 , 1319–1333.
Yoganathan, S., Finch, D. A., Parkin, E., & Pollard, J. (2018). 360° virtual reality video for the acquisition of knot tying skills: A randomised controlled trial. International Journal of Surgery, 54 , 24–27.
Download references
Author information
Authors and affiliations.
Division of Psychology, School of Education & Social Sciences, University of the West of Scotland, Paisley, UK
D. Hamilton, J. McKechnie, E. Edgerton & C. Wilson
You can also search for this author in PubMed Google Scholar
Corresponding author
Correspondence to D. Hamilton .
Additional information
Publisher's note.
Springer Nature remains neutral with regard to jurisdictional claims in published maps and institutional affiliations.
See Table 2 .
Rights and permissions
Open Access This article is licensed under a Creative Commons Attribution 4.0 International License, which permits use, sharing, adaptation, distribution and reproduction in any medium or format, as long as you give appropriate credit to the original author(s) and the source, provide a link to the Creative Commons licence, and indicate if changes were made. The images or other third party material in this article are included in the article's Creative Commons licence, unless indicated otherwise in a credit line to the material. If material is not included in the article's Creative Commons licence and your intended use is not permitted by statutory regulation or exceeds the permitted use, you will need to obtain permission directly from the copyright holder. To view a copy of this licence, visit http://creativecommons.org/licenses/by/4.0/ .
Reprints and permissions
About this article
Hamilton, D., McKechnie, J., Edgerton, E. et al. Immersive virtual reality as a pedagogical tool in education: a systematic literature review of quantitative learning outcomes and experimental design. J. Comput. Educ. 8 , 1–32 (2021). https://doi.org/10.1007/s40692-020-00169-2
Download citation
Received : 21 October 2019
Revised : 16 June 2020
Accepted : 02 July 2020
Published : 11 July 2020
Issue Date : March 2021
DOI : https://doi.org/10.1007/s40692-020-00169-2
Share this article
Anyone you share the following link with will be able to read this content:
Sorry, a shareable link is not currently available for this article.
Provided by the Springer Nature SharedIt content-sharing initiative
- Virtual reality
- Head mounted displays
- Simulations
- Find a journal
- Publish with us
- Track your research
Virtual Reality in Education: Benefits, Tools, and Resources
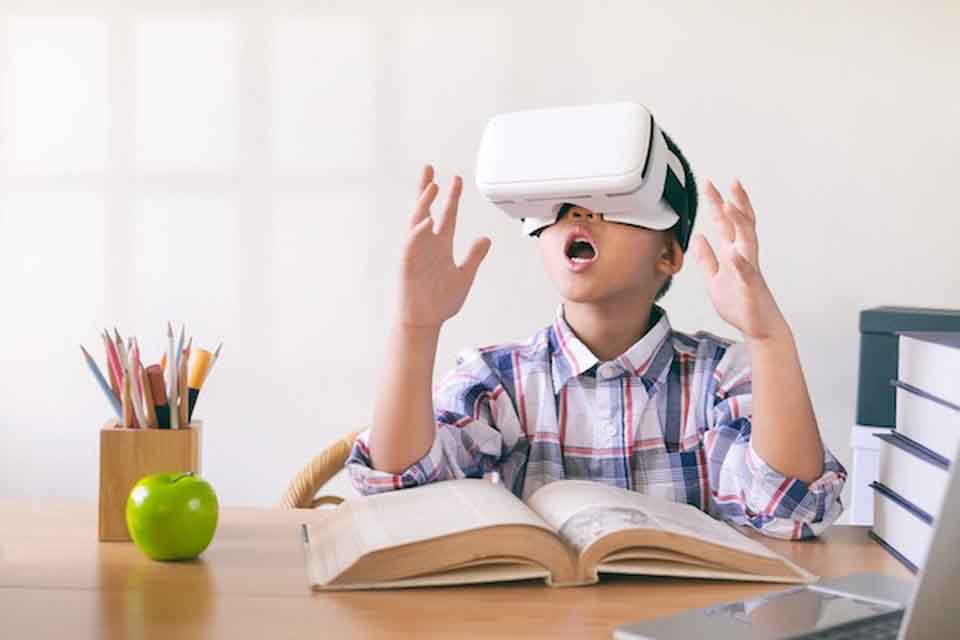
In the 1966 film Fantastic Voyage , a submarine and its crew shrink to the size of a human cell to ride through the bloodstream of a scientist and remove a blood clot in his brain. An imaginative tale of science fiction, the movie speaks to humanity’s desire to explore realms considered impossible to reach due to our physical limitations. But thanks to technologies such as virtual reality (VR) and augmented reality (AR), students in elementary schools are now doing just that. Today, students go on virtual field trips to places ranging from the Roman Colosseum in ancient times to outer space to cellular-level passageways inside the human body.
The benefits of virtual reality in education are embraced by many educators, but some are still reluctant to use it in their classrooms. Reasons range from high costs to pushback from school administrators. Others see the value of both VR and AR as entertainment, but not as effective teaching tools in the classroom. Additional educator concerns, as reported in a recent EdTech report, include the bulkiness of the equipment, glitches, and the quality and availability of content. Despite these challenges, demand for AR and VR in education is expected to grow in the coming years. This means that current and aspiring teachers should take steps to learn about the benefits of virtual reality in the classroom.
Innovative teacher education programs like American University’s Master of Arts in Teaching help graduates become forward-thinking educators who can inspire students through technology. The program’s focus on preparing graduates with the skills to deliver education using a multidisciplinary approach is especially helpful.
The program prepares graduates with real-world technical skills using advanced virtual platform technologies. “The use of Mursion [VR] technology has provided American University’s teacher candidates the opportunity to practice science instruction before they work with ‘real’ students, enhancing our teacher candidates’ confidence and skill,” says Carolyn Parker, director of the Master of Arts in Teaching program in American University’s School of Education.
What Are the Benefits of AR and VR in Education?
Before looking into some of the benefits of virtual reality in education, let’s define what virtual reality is and how it differs from augmented reality. AR is used on a smart device to project a layer of educational text and lesson-appropriate content on top of a user’s actual surroundings, providing students with interactive and meaningful learning experiences. VR creates an entire digital environment, a 360-degree, immersive user experience that feels real. In a VR setting, students can interact with what they see as if they were really there.
In addition to providing students with immersive learning experiences, other benefits of virtual reality in education include the ability to inspire students’ creativity and spark their imaginations. And this can motivate them to explore new academic interests. AR and VR in education also helps students struggling to understand difficult academic concepts. For example, through AR, geometry students can check out 3D geometric forms from multiple perspectives; they can rotate a shape to see it from different angles and even view it from the inside. The benefits of virtual reality in education go beyond academics as well to include cultural competence, the ability to understand another person’s culture and values—an important skill in today’s interconnected, global society. For example, a virtual reality field trip to other parts of the world, whether it be Peru or China, exposes students to cultures other than their own.
Growing evidence suggests that AR and VR in education, as well as the combination of both technologies known as mixed reality, can improve student outcomes, too. For example, in a March 2019 report, EdTech cites a study showing that students in a mixed reality biology classroom received higher scores than other students. And AR and VR can help with memory retention and recall, as well—EdTech reports on a recent study that shows an increase in retention of almost 9 percent for students who learned in an immersive environment such as VR.
AR and VR in Education: Resources and Tips
Bringing AR and VR tools into the classroom doesn’t have to be expensive. Available resources, ranging from low-priced viewers like Google Cardboard to cost-effective equipment that can connect to smartphones, can be acquired without breaking the bank. Resources for teachers include affordable or even free apps, such as 360Cities, which allows students to visit places like Rome and Tokyo. Another app, TimeLooper, allows students to visit locations through a historical lens, such as London in medieval times or World War II. Platforms like Immersive VR Education and Nearpod allow teachers to develop lesson plans with VR and AR technology.
These, and other resources, are key to incorporating immersive education into classrooms. But how can teachers set up their classrooms to maximize the benefits of VR in education? Here are a few tips.
Ensure Ample Physical Space
To reap the benefits of virtual reality in education, it is important for students to use VR equipment safely. VR users often spin around or stride blindly, ignoring their physical surroundings. A misstep could lead to injury. Educators should ensure their classrooms’ physical environments are spacious and safe for VR explorers. Students should be at least an arm’s length away from each other and from objects in the classroom. When possible, use VR content that can be accessed by students sitting at their desks.
Supervise and Moderate VR Use in Classrooms
Research into the psychological impact of VR on students suggests that VR should be used moderately and under close supervision in school settings. The findings of the research as reported in a recent CNN.com article recounts that children who overused VR had false memories of having physically visited a place they actually never visited. Limiting VR education sessions to a couple of minutes as part of a longer lesson plan can address this issue.
Know When to Use VR in the Classroom
VR can bring academic subjects to life, offering students new insights and refreshing perspectives. But VR can’t replace human interaction. Learning is fundamentally a social experience, so VR is best used as a supplemental learning tool.
How can teachers use VR in the classroom? It depends on the subject. Using VR to teach grammar in classrooms may not make much sense because grammar is a relatively abstract topic. On the other hand, VR may work well for topics that are visual and tactile, for example, allowing students to learn “firsthand” about a historical event or famous monument.
As a case in point, because the Parthenon in Greece is a physical structure, students can virtually walk inside it to see its architectural details, thanks to VR equipment and software. Many STEM (science, technology, engineering, and math) topics also lend themselves well to VR. When it comes down to it, what child wouldn’t enjoy “visiting” the planets of the solar system?
Develop a Plan for VR Learning
Among the most noteworthy benefits of virtual reality in the classroom is its ability to spark curiosity and interest in students. But left to their own devices, students may veer off topic. This is why educators should develop a structured plan to maximize the use of VR within lesson plans and then guide their students along the path. As part of the plan, it is important for teachers to determine goals and expectations for students and set guidelines for students to follow to ensure optimal learning experiences.
Teach Empathy and Cultural Competence
The magic of VR is that it brings different places throughout the world right into the classroom. These new perspectives can result in fostering empathy and cultural competence because they take students outside of their normal daily experience. The use of VR and AR helps students understand people’s unique situations across the world. For example, teachers can use VR applications to enhance language teaching by exposing students to the cultures of the people who speak the language. Using technology to build culturally responsive environments helps students respect cultures different from their own.

Become an Innovative Education Leader
Innovative educational technologies such as VR and AR continue to emerge. And according to an April 2019 EdWeek report, 15 percent of US schools will have classroom access to virtual reality by 2021. How can teachers prepare to leverage the benefits of VR in education to improve learning outcomes?
With a focus on offering future teachers the essential tools needed to transform the education system and educate all students equally, American University’s Master of Arts in Teaching program equips graduates to become innovative education leaders. Advances in neurodevelopment, social-emotional interventions, and a multidisciplinary approach to teaching STEM education are central to the program’s curriculum so that students develop skills to succeed in an education field driven by new technologies such as VR and AR.
If you are looking to advance your education career by leveraging technology, learn how American University’s Master of Arts in Teaching program can help put you at the forefront of the educational innovations that are transforming schools and lives.
Classroom Tips for Success: Valuable Teaching Skills to Master
How to Be a Better Teacher: Reaching Students in the 21st Century
The Future of Education Technology (Infographic)
CNN, “Can Virtual Reality Revolutionize Education?”
Common Sense, Top Tech for Using Augmented and Virtual Reality
Common Sense, “What the Research Says About VR in Classrooms”
EdTech, “3 Exciting Ways to Use Augmented and Virtual Reality in the K–12 Classroom”
EdTech, “K–12 Teachers Use Augmented and Virtual Reality Platforms to Teach Biology”
EdTech, “Survey: Education Among Top Industries for AR/VR Investments”
Education Week, “A Global Perspective: Bringing the World Into Classrooms”
EdWeek Market Brief, “Education Seen as Strong Market for VR and AR by Industry Insiders”
Getting Smart, “Building Culturally Responsive Classrooms with Digital Content”
IMDb, Fantastic Voyage (1966)
International Society for Technology in Education, “25 Resources for Bringing AR and VR to the Classroom”
Medium, “Augmented Reality and 3D Geometry — Bring Some Magic to Your Classroom”
THE Journal, “Making Virtual Reality a Reality in Today’s Classrooms”
Request Information
- Open access
- Published: 19 October 2020
Exploring the trends of educational virtual reality games: a systematic review of empirical studies
- Solomon Sunday Oyelere ORCID: orcid.org/0000-0001-9895-6796 1 ,
- Nacir Bouali 1 , 2 ,
- Rogers Kaliisa 3 ,
- George Obaido 4 ,
- Abdullahi Abubakar Yunusa 5 &
- Ebunayo R. Jimoh 6
Smart Learning Environments volume 7 , Article number: 31 ( 2020 ) Cite this article
26k Accesses
52 Citations
15 Altmetric
Metrics details
Virtual Reality (VR) and educational games are emerging technologies mediating a rapid transformation in the educational world. However, few studies have systematically analyzed Educational Virtual Reality Games (EVRGs) and how they have been applied in educational settings. This study reviewed 31 articles published in high impact journals and educational conference proceedings to unravel the technological, pedagogical, and gaming characteristics of contemporary EVRGs. The results show the predominance of Oculus Rift headsets and HTC Vive as the main technology used in EVRGs. Moreover, the analysis revealed that the pedagogical application of the majority of EVRGs was developed for all levels of education (e.g. tertiary, K-12, lifelong learning), with the specific target audience of each game based on the desired learning outcome. Furthermore, the application of EVRGs has primarily focused on out of class use, with healthcare education topics dominating the topics taught using EVRGs. Based on our findings, we highlight some key implications and suggestions to advance the field of EVRGs.
Introduction
This study explores the advances of educational virtual reality games (EVRGs) and expounds its important developmental features such as technology, pedagogy and gaming. The rapid development in Information and Communication Technology (ICT) has revolutionized the computing industry and propelled a paradigm shift in the pedagogy of teaching and learning (Kaliisa, Edward, & Julia, 2019 ; Oyelere, Suhonen, Wajiga, & Sutinen, 2018 ). Contemporary computer hardware and software have improved significantly in size, speed, and precision, and a key to the creation of immersive technological applications (Bekele, Pierdicca, Frontoni, Malinverni, & Gain, 2018 ; Voinea, Girbacia, Postelnicu, & Marto, 2018 ). Virtual reality (VR) is a technology that has recently gained prominence as one of the core features of modern ‘high-tech’ with wide-ranging applications, including education (Virvou & Katsionis, 2008 ), gaming (Zyda, 2005 ), entertainment (Liu, Cheok, Mei-Ling, & Theng, 2007 ), military (Lele, 2013 ), skills training (Aggarwal, Black, Hance, Darzi, & Cheshire, 2006 ), tourism (Tussyadiah, Wang, Jung, & Dieck, 2018 ), as well as physical exercise (Finkelstein et al., 2011 ). VR is computer-simulated, which gives users the illusion of being physically present in the world and uses not only sight but also sound and touch to fully engage a user in the virtual world (Mandal, 2013 ).
In this paper, we refer to VR as the experience in which a user is fully immersed into either a virtual environment using head-mounted displays (headsets) or projection-based displays. A user through the utilization of an avatar (Carvalheiro, Nóbrega, da Silva, & Rodrigues, 2016 ) can as a result, navigate this world. This functionality differs from 3D environments visualized using headsets, but the user is only able to experience the virtual world from a fixed perspective and an onscreen visualizable three-dimensional (3D) environment. In this research, educational games are defined as the games designed, implemented, and evaluated with the purpose of teaching, or aiding in the instruction of a subject, or aiding in the learning of a specific skill within a formal or an informal setting (Oyelere, Suhonen, & Laine, 2017 ; Pavlidis & Markantonatou, 2018 ). We define Educational Virtual Reality Games (EVRGs) as educational games that exploit the 3D stereoscopic display, using a wearable headset or a Cave Automatic Virtual Environment (CAVE) system to teach or aid in the instruction of a specific topic. With such a definition non-immersive educational VR games, wherein the user navigates a virtual world using an avatar controlled by a mouse and a keyboard and/or a joystick, have been excluded.
VR technology has become increasingly a popular teaching and learning support tool across different disciplines. It provides an opportunity for students and teachers to experience, as well as interact, with real-time learning phenomena, something that would have been almost impossible in the physical world (Shin, 2017 ; Vesisenaho et al., 2019 ). VR allows for the use of multiple senses (e.g., touch, sense of heat, smell), which are used simultaneously during the learning process. In this regard, this could improve the activeness and mental alertness of both the students and teachers. This claim is supported by Lee and Wong ( 2014 ) who concluded that there is a significant interaction effect between the learning mode and the spatial ability of the students. Furthermore, other studies have established the pedagogical benefits of VR, such as the ability to support students with diverse learning styles in gaining cognitive achievement (Lee, Wong, & Fung, 2010 ), improving spatial thinking (Cohen & Hegarty, 2014 ), learning object-oriented programming concepts (Bouali, Nygren, Oyelere, Suhonen, & Cavalli-Sforza, 2019 ), and in facilitating collaboration (Greenwald et al., 2017 ).
The goal of this study was to systematically review the trends of EVRGs from the perspective of their technological, pedagogical, and gaming characteristics. The study draws on research conducted by Laine ( 2018 ), by focusing on studies that describe the design, implementation, and evaluation of EVRGs. Since Laine’s ( 2018 ) study focuses strictly on mobile Augmented Reality Games (ARs), our work provides a slightly different and more comprehensive perspective by focusing on EVRGs. A review of previous studies of EVRGs would make it possible to understand in which direction the field is heading and how future EVRGs should be designed to best fit the needs of both the teacher and students to improve educational outcomes. We argue that a review of EVRGs is needed (i) to understand and conceptualize the existing body of EVRGs; (ii) to provide evidence about the technologies that support the implementation of EVRGs across a wide range of settings and techniques used; (iii) to explore the teaching and learning attributes of VR games and how different pedagogical characteristics such as context, topics taught, and types of learners have changed over the years. We further aim (iv) to develop a set of pointers to researchers and practitioners (e.g. teachers) interested in conducting and applying VR games in research and pedagogical practice; and (v) to understand the characteristics of contemporary games to supporting educational developments. To achieve these goals, we, therefore, seek to answer the research question: What are the technological, pedagogical, and gaming characteristics of contemporary educational VR games?
The rest of the paper is organized as follows: presentation of related work, the methodology, and conclusion with several remarks that reinforce the critical points regarding the use of educational VR games.
Related work
Relationship between vr and education.
The educational process requires the learner to grasp and comprehend abstract concepts and appreciate scenarios, as well as to understand situations which are far removed from the confines of the classroom. Duffy and Jonassen ( 1992 ) argue that the teaching of abstract phenomena, analogy and lived experiences are used to describe and ease through abstract concepts from commonly observable reality. However, over the last decade, the application of emerging technologies in education have revolutionized the pedagogical or teaching processes in the classroom, to explain better and to provide comprehension of abstract concepts.
VR is an emerging technology that has gained traction in education. Educationists have discovered that VR allows the user to interact with a computer-generated 3D model or virtual environment (Christou, 2010 ) which fosters the understanding of the imaginary world on a realistic scale. This makes it a useful tool for teaching and learning by transforming the way educational content is delivered (Hentsch, 2018 ). A study by Lee and Wong ( 2008 ), described VR as a technology that aids student learning through the visualization of information and engagement, which affords a learner with the opportunity to experience subject matter or concepts that are not easily discernible.
VR does not only relate positively to education but aligns with the constructivism school of thought, which stipulates that humans construct knowledge by learning from experience. The constructivist theory advocates that learning through interaction with sensory data allows knowledge construction from experience for which VR is suited. Christou ( 2010 ), chronicled broad application areas of VR in educational contexts used in the enhancement of core curricula in schools and colleges, application of VR in museums, edutainment, demonstrations, simulation, and training.
Comparison of VR and 3D games in education
In the past few decades, game-based learning has been an integral part of the educational process (Boboc, Orzan, Stoica, & Niculescu-Ciocan, 2018 ; Hwang, Wu, Chen, & Tu, 2016 ; Xenos, Maratou, Ntokas, Mettouris, & Papadopoulos, 2017 ). EVRGs are designed to assist users to grasp the concepts of a specific subject, to expand their knowledge, and to facilitate participation. A typical example of educational virtual reality game is GridlockED, which was developed by Tsoy et al. ( 2019 ) as a collaborative learning game, targeted for medical trainees, to acquire the skills on how to treat and triage a patient. Modern educational games are played using high-end video technology such as stereoscopic 3D, or through a Head-Mounted VR environment (Snowdon & Oikonomou, 2018 ). These technologies use a spatial depth on the screen and offer rich learning experiences for the user.
A study by Sampaio, Ferreira, Rosário, and Martins ( 2010 ) described 3D technology as that which creates spatial depth with 3D pop-up visualizations so that objects contained in the game may appear closer to the player with the use of dedicated 3D-capable glasses. In comparison to the 3D technology, Triberti, Villani, and Riva ( 2016 ) described VR as a computer-simulated reality that uses a 3D environment whereby the player interacts with using a specialized head-mounted display (e.g. Oculus Rift, Samsung Gear VR, Google Cardboard etc.) that shows visual effects to the eyes. Unlike 3D, where the user is a just a viewer, VR allows for the user to become part of a story, offering an untethered immersive experience (Bradshaw, 2016 ). Therefore, a video game in VR is more realistic than one played in a 3D condition. Hence, VR technology could be applied to complement 3D modelling, ensuring better communication both in an educational or vocational training (Bradshaw, 2016 ). A comparative study by Roettl and Terlutter ( 2018 ) on 237 players showed that the participation and cognitive load was much higher in players using VR than just 3D. Recently, researchers have critically debated on the effects of 3D and VR technologies on issues such as adverse effects like social isolation (Nicas, 2018 ), discomfort, eye fatigue, and headache when using these technologies for an extended period (Bradshaw, 2016 ; Roettl & Terlutter, 2018 ; Sharkawi, Ujang, & Abdul-Rahman, 2008 ).
Related EVRGs reviews
Since this article focuses on exploring current EVRGs, we consider it vital to summarize in a tabular form the existing efforts in this direction. Table 1 presents the previous attempts to review works in EVRGs.
As can be observed from the existing studies reviewing EVRGs (Table 1 ), the field of VR games has garnered interest amongst educational technology researchers. This may be partly as a result of an increase in innovation within the field as well as in the variation in the pedagogical application of VR. However, to the best of our knowledge and evaluation of the existing reviews, no study has attempted to review systematically the trends of EVRGs accounting from the viewpoints of technology, pedagogy, content, knowledge, and games. To bridge this gap, this paper centers in a systematic literature review to examine the current research on EVRGs and to provide important insights beyond the specific research findings within the individual studies.
Methodology
This study follows the guidelines for conducting a systematic literature review by Kitchenham and Charters ( 2007 ) (Fig. 1 ). The search service of the (blinded for review) library’s printed and electronic resources, (blinded for review) was utilized to access databases and extract publications. Google Drive was used for collaboration among the team, and MS Excel spreadsheets were used to manage and organize the information acquired from the search.
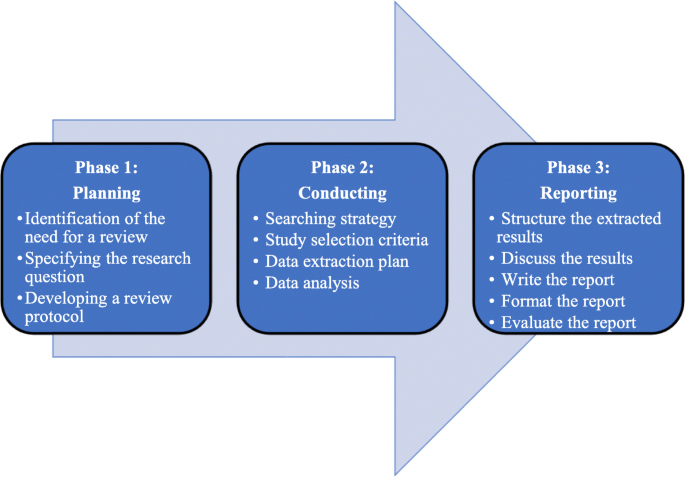
The systematic literature review process based on guidelines by Kitchenham and Charters ( 2007 )
Phase 1. Planning the review
This phase focuses on the initial preparation undertaken to achieve the goal of this literature review.
Phase 1.1 Rationale of the review
Based on the guidelines by Kitchenham and Charters ( 2007 ), we identified previous systematic reviews that addressed either our research questions or similar questions. However, as discussed in the introduction, none of the reviews focused on EVRGs. Thus, we decided to conduct a review on EVRGs with specific attention to attributes such as technology, pedagogy and gaming.
Phase 1.2. Specifying the research question
By drawing on existing accounts about the application of VR games, in the educational sphere, this study set one research question: What are the technological, pedagogical, and gaming characteristics of contemporary educational VR games? To answer this research question, this study analyzed existing research about EVRGs with an emphasis on profiling the games in terms of the country of research, year of production, technological attributes (e.g. platform, types of headsets), pedagogical attributes (e.g., topics, types of learners, educational settings) and the gaming attributes (e.g., player’s role, the theme of play, mode, and goal of the game).
Phase 1.3. Developing a review protocol
According to Kitchenham and Charters ( 2007 ), a review protocol provides the basis to carry out the systematic review. Creating a review protocol beforehand helps to reduce the likelihood of research bias, such as potential prejudiced selections of particular studies carried out by the researcher. Formal and informal searches were used to find relevant studies in response to the research question. Table 2 presents the databases and the search strategy of the preliminary searches of existing related studies of EVRGs in education, which set the direction for this study. The databases were selected because they were either specialized in educational technology, educational games or in virtual reality applications, or that have published at least one special issue in educational virtual reality and games for learning. Thereafter, the preliminary review studies presented in Table 1 were used to create the research framework as well as the research question, which yielded a well-organized review protocol.
Phase 2. Conducting the review
The second phase of the guidelines by Kitchenham and Charters ( 2007 ) follows five stages: the search strategy, the study selection criteria, the study quality assessment, the data extraction plan, and the data analysis tool.
Phase 2.1. Search strategy
The research question of this study guided the formulation and expounding of the search strategy. As a first step, the search keywords were identified as a way of narrowing down and focusing on relevant articles for the topic under study. The search keywords were chosen according to the research theme; research question and the objective of this study (see Table 2 for search strategy and list of databases). Search keywords: The search space was narrowed down using Boolean search phrases and different combinations of the following terms: “virtual reality” AND “education”, OR “learning”, OR “game”, OR “gamification”, OR “serious game”. While many researchers use the term “educational games” to refer to games, the purpose of which is helping a learner acquire a skill, others opt for a more generic term like “serious game”. The keyword choice was made in a way to capture any game that is used in an educational context, be it formal or informal, as long as it uses VR technology, with the definition we adopted above. The time frame for publication considered within the systematic review was between 2012 and 2018 . Since the field of educational technology and virtual reality games is developing very fast, articles before 2012 have been reviewed in previous studies and are not particularly relevant in this work. The screening was based on titles, abstracts, and full-text skimming and took place from 1 February 2019 to 30 May 2019.
Phase 2.2. Study selection criteria
Using the keywords and search strings presented in the section “ Phase 2.1. Search strategy ”, several articles were selected based on their relevance to the research question and inclusion criteria. A flow diagram representing the steps of the selection criteria is presented in Fig. 2 . The researchers read the title, abstract, keywords and skimmed through the contents of all the papers and selected the articles that appeared to be appropriate based on VR, education, and gamification. Abstracts, posters, books, and articles that did not show an implementation that required a VR headset were excluded. Altogether, we found 162 research papers through the predefined search keywords on the four databases. However, after we applied the inclusion and exclusion criteria (Table 3 ), only 31 articles made it through the scrutiny (Table 4 ).
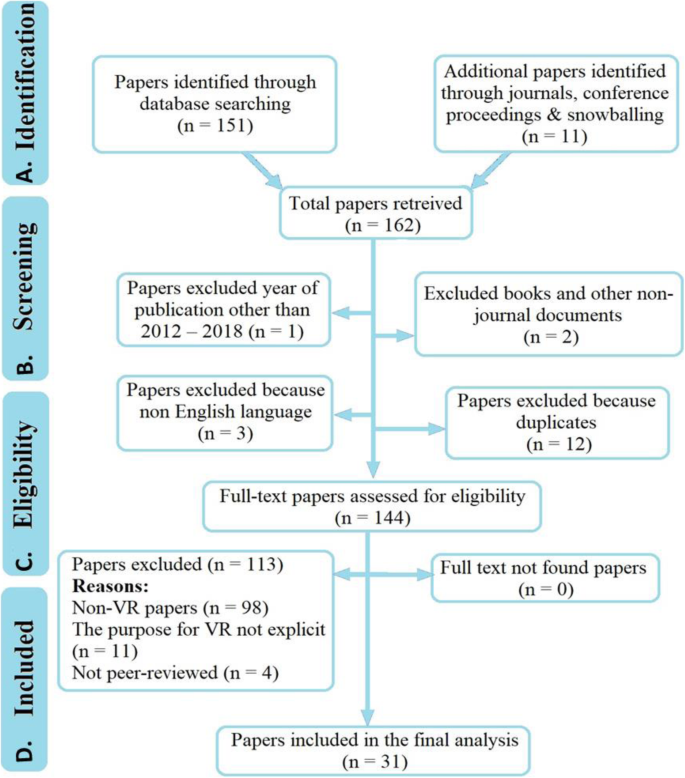
Flow Diagram of the Review Process (Adapted from Moher, Liberati, Tetzlaff, & Altman, 2009 )
Phase 2.3. Data extraction and analysis
We set up a coding scheme to guide the extraction of relevant data from research articles with relevance to our research question. The coding scheme included the following overarching dimensions: Title/name of the game, description of the game, country of game implementation, player role, theme, mode, gameplay, goal, platform, VR headset, game interaction, focus, learners, educational setting, research, and evaluation methods.
In summary, the methodology section, presented the planning and implementation process of the systematic review and these included; providing the rationale for choosing the framework adopted for the review, specifying the research questions, developing the review protocol, executing the review, identifying the keywords, drafting the study selection criteria as well as data extraction and analysis. Subsequently, the results yielded by these processes are presented in the next section.
The results are organized in four main categories: A general overview of the EVRGs, technological, pedagogical, and gaming characteristics.
Overview of the VR serious games
The number of EVRGs publications appearing in each year from 2012 to 2018 is presented in Fig. 3 . The bar chart shows a definite increase in the number of research works dedicated to VR educational games. Research shows that there was one study in 2014 and increases to 14 studies in 2018. Table 5 shows that EVRGs have been researched in North and South America, Europe, Asia, and Australia. See Table 5 for full details of serious games.

The number of research publications dedicated to VR games per year
Technological characteristics
The review looked at the technological characteristics of the selected EVRGs in terms of platform, headset, and interaction components. As shown in Fig. 4 , the Oculus Rift seems to dominate the VR headsets used in EVRGs, as it was used in nearly half of the games (45.2%). HTC Vive came in second used in almost a quarter of the games (22.6%). Cardboard, which is the cheapest of the VR headsets, was only used in 9.7% of the games, while under a quarter (22.6%) of the papers we reviewed, did not specify which headsets were used as target technology.
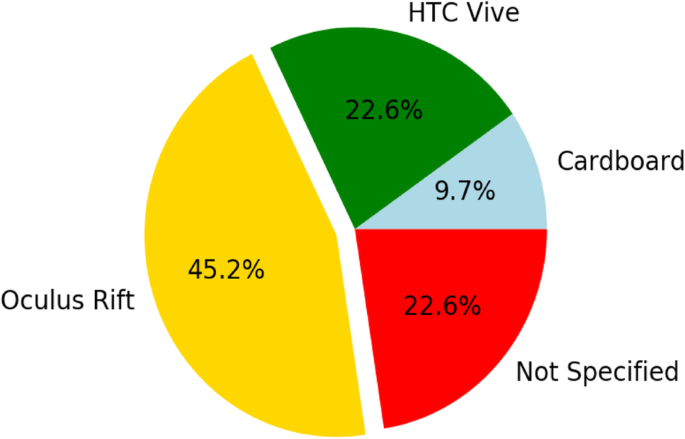
Headsets breakdown in surveyed literature
The platforms used for the games were consistent with the headsets, given that HTC Vive and Oculus Rift DK2 are PC VR devices, 83.9% of the games were playable using a PC or MAC, while 12.9% were playable using a mobile device, which matches the Cardboard requirements (Fig. 5 ).
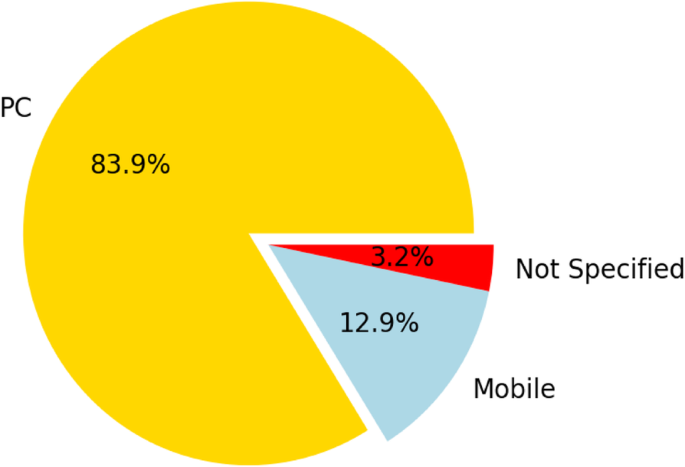
The distribution of the platforms in the literature within the SR
In Table 6 (technological characteristics), we show the interaction techniques and hardware used in the EVRGs. While the most basic headsets (e.g. Cardboard) provide basic VR interaction mechanisms like gaze and head movements, more advanced headsets come with more sophisticated controllers. These controllers allow the users to interact with the props in the game environment and facilitate movement. Some games provide natural user interfaces, while other task-specific games provide more advanced controllers such as steering wheels. However, it is noteworthy that traditional input devices, such as a mouse or keyboard are challenging to use with VR technology, as the latter conceals the user in a virtual environment, disallowing the visibility of the surrounding real environment. Some games have, however, suggested such input devices, which makes the gaming experience slightly inconvenient.
Pedagogical characteristics
The analysis of the games showed that more than two-thirds of the educational VR games were developed for informal learning context (Fig. 6 ). While the topics taught in the analyzed games were of great variety, we tried to group them under specific themes, to understand which of the tasks or learning goals were deemed VR-appropriate by the researchers. For instance, one-third of the games targeted teaching healthcare-related topics, while a quarter of the games aimed at introducing, training, or enforcing safety measures in various environments (e.g., construction sites, hospitals, or roads). Topics such as biology, physics or astronomy represent a tenth of the surveyed games (represented in Fig. 7 as natural sciences). While topics like language learning, geography, and civil engineering, received minimum interest with one VR game each.
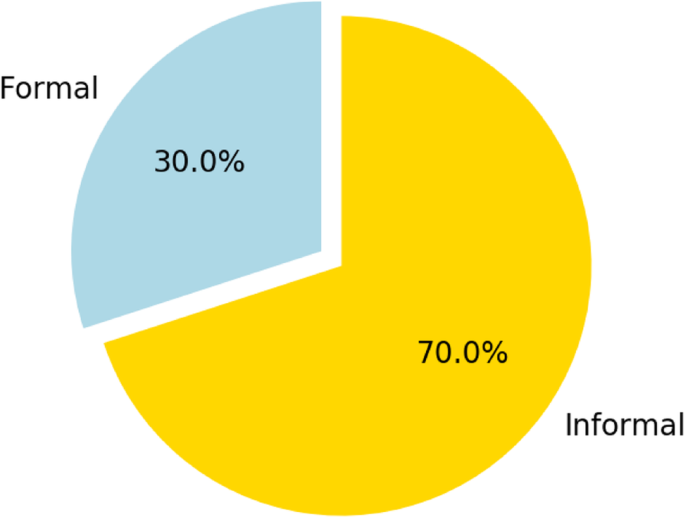
Game settings
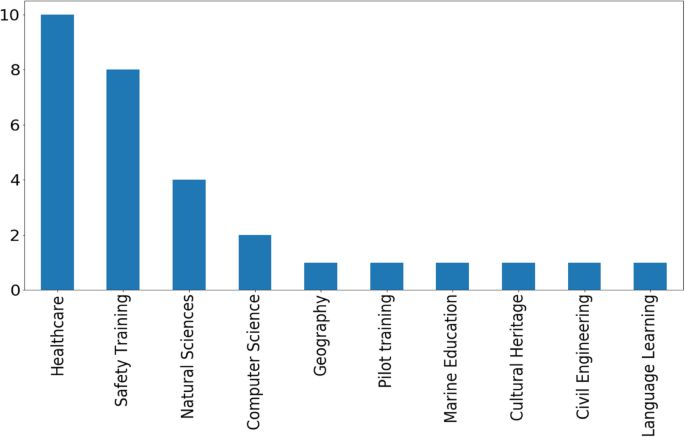
The topics taught using VR educational games
The target audience of the EVRGs depended on the desired learning outcomes. Additionally, the VR games were developed for all levels of formal education, from K-12 to tertiary education students, as well as for lifelong learners who need to acquire some skills to deal with specific health conditions, as illustrated in Table 7 .
Gaming characteristics of EVRGs
The 31 EVRGs reviewed in this paper differ significantly in the player roles suggested to their users but are mainly dependent on the educational purpose of the tool. Most of the games suggest player roles that are adequate for the tasks the user is being prepared for, in environments similar to those that they will be working on (see Table 8 for several aspects of gaming characteristics). This shows the power of VR in providing learners with a preview of working activities and conditions.
VR facilitates collaborative learning, where many learners can be together in the same virtual world to execute some learning tasks. Only five EVRGs incorporated such a feature in their designs, while 26 games decided to allow only single-player mode. Gameplay-wise, most games suggest the user moves through the virtual world and executes tasks similar to the real-world tasks they are being prepared for, and so games genres like puzzles are quasi-inexistent in the game set that we have reviewed. This study has found that the VR games present the content to be taught as a series of entertaining challenges to the learner in a virtual environment.
General overview
The study revealed that the number of articles or literature on emerging VR systems has been increasing since 2012, which indicates the interest VR has gained since its spread in late 2012. The study also revealed many educational topics in which VR has already been applied. A general emphasis was placed on healthcare education by VR researchers, along with a variety of other topics such as biology, computer science, astronomy, and fire training (Sárkány, 2016 ; Süncksen et al., 2018 ). VR has the potential to allow users to experience environments that are otherwise inaccessible in a very realistic way. It allows training in environments that would otherwise be hazardous for learners to train in, as is the case for fire training (Diez et al., 2016 ). It also allows learners to simulate training with expensive hardware on a risk-free yet realistic environment. Despite these affordances and the application of VR unraveled by this study, there appears to be a lack of research on the use of VR for language learning, failing to benefit from the technology in simulating social interactions, which are very efficient in helping learners to practice their language skills effectively.
Another key finding of this research is the absence of any VR educational games on the African continent. While North and South America, Europe, Asia and Australia participated in developing EVRGs. The cost of technology seems to affect African countries from benefiting from this promising technology. One solution is to rely on affordable headsets supported by Google Cardboard (Bouali et al., 2019 ). Still, such headsets suffer from the lack of appropriate interaction hardware that allows users to traverse and interact with the virtual world using only gaze and head movement. Such limitation causes Cardboard-related research to engineer games that require minimum interaction while preserving the targeted learning outcomes.
Technology and gaming
Despite Cardboard being the cheapest VR device, it is the least used technology in the surveyed literature, ranking even lower than the expensive HTC Vive. This raises some questions on the level of adoption of some educational games in real educational contexts, which is not the focus of our study. Nevertheless, the study shows that various games have adopted the use of the Oculus Rift VR, despite being more expensive than Cardboard headsets. Consequently, headsets are not the only problem in VR adoption. This study reveals that interaction is also an issue. In our analysis, we found that most of the games rely on gaze as an interaction mechanism, but this is hardly ever complemented with a natural user interface or interfaces, which facilitate interactions in VR worlds, resulting in the difficulty of using other input devices like keyboards.
Games that target teaching specific skills, such as diving or driving, require more advanced input devices, like Kinect to input body movement or a steering wheel to provide a life-like controller to a vehicle in the virtual world (Calvi et al., 2017 ; Likitweerawong & Palee, 2018 ). However, this incurs that such technology can only be afforded by a handful of users, presenting a stumbling block in the adoption of VR games in real-world contexts.
Regarding gaming, most of the gaming environments and contexts were dependent on the real-world context for which the learner is being prepared. This helps in the learning process of the user as it provides him/her with tailored environments that mimic the real world to a higher level of detail. The study also reveals that most of the games developed, allowed only for a single-player mode, failing to benefit from VR’s ability to connect learners in virtual worlds. The dominant game genre in the literature is Role Playing Game (RPG), which is adequate given that the learner impersonates the roles they are being trained for in the virtual world.
The concept of applying digital technology to build a virtual, physical/virtual or hybrid learning environment in which a student experiences a form of play, has emerged over the years and is now gaining acceptance. The puzzle here is that despite the wide variety of VR games developed for different fields (education, healthcare, business, climate, energy, industrial, and financial) only a handful of the games focused on education. Thus, begging the question of why EVRGs have not been widely adopted in mainstream education? Considering the interactive, immersive, and multi-sensory nature of VR, coupled with its growing popularity amongst researchers in psychology, aviation, and cognitive neuroscience, we had expected that even more EVRGs could have been developed and that the technology should have been widely adopted (Alexander et al., 2019 ). However, there are many reasons for the lack of adoption of EVRGs in mainstream education. One reason is that people tend not to consider VR as a mainstream technology. They perceived that the hype around the technology would lose popularity and be replaced by the reality of the time, in what was called the ‘trough of disillusion’ (Linden & Fenn, 2003 ). Additionally, there seems to be a lack of know-how by learning technologists and experts on how to design learning solutions with VR. Furthermore, the costs of implementing EVRG for large-scale adoption across educational curricula constitute a limiting factor for adoption in mainstream education (Alexander et al., 2019 ) and health-related issues.
Christou ( 2010 ) states that the three categories of formal and informal educational application areas of VR are used to improve core curriculum subjects such as the applications for edutainment, demonstrations, cultural heritage and museum experiences, as well as an application for training. Our analysis of the pedagogical contributions of EVRGs indicated a considerable variation among the subjects that were implemented. For example, the trend of EVRGs in this study showed that 32% of the applications were developed to support medical education. In contrast, only 3% was implemented to support subjects that involve the concretization of abstract concepts such as physics and geography.
Although the learners in these studies balanced well amongst children, youth and adult learners, there are obvious variations amongst the type of learners about the kind of EVRGs. For example, most learners could have medical conditions such as patients with neck or back pain, dementia, autism spectrum disorders, post-stroke conditions, brain lesions, and visual impairment. Translating clinical procedure into EVRGs to facilitate learning and future implementation of the procedure by caregivers and patients constitute the main interest in developing medical EVRGs (Heuven et al., 2017 ; Mihajlovic et al., 2018 ). Simulating the medical procedure offer motivation, engagement, and positive learning experience among EVRGs users (Trombetta et al., 2017 ). Having examined an essential pedagogical attribute, of the context of the learner in this study, we noticed that the learning context for most EVRGs falls within the informal settings. As several EVRGs are developed to provide particular training solutions to the user, the informality of the setting tends to overshadow the necessity of a classroom environment (formal setting).
Limitations of this study
The limitations of this study include:
The study focused on EVRGs in the educational domain without considering other uses in other facets within the gaming industry.
The study did not consider cross-cultural usage of the EVRGs, such as articles published in languages other than English.
Several studies have shown that the most critical value that VR adds to existing technology is the sense of immersivity in a virtual world built around the user. In education, it is theoretically crucial for learners to experience real-world scenarios from a first-person perspective compared to traditional two-dimensional (2D) screens which usually offer learners the chance to traverse the world from a third-person perspective, usually using an avatar. “Virtual Reality” as a concept, reveals some ambiguity as some of the research we surveyed referred to it as desktop VR, while others use the term to mean immersive VR. However, the inclusion criteria we developed focused on working on systems that immerse users into a virtual world rather than research that suggests a virtual world on a desktop screen.
Virtual reality, augmented reality, and mixed reality are rapidly evolving phenomena in the educational landscape. Literature has shown the positive impacts that these adaptive and immersive technologies could have on students learning when applied in the gaming contexts. The essence of this study was to contribute new knowledge using analysis and synthesis of research articles that focused on the EVRGs in different contexts. The study explores the trends of EVRGs and relevant characteristic attributes that make effective learning such as technology, pedagogy and gaming. Besides, this study considers the learning content and the technology to be the critical aspects of the transactions that go with the teaching and learning process in terms of the pedagogy.
Moreover, the review focused on the application of VR as a gaming technology, with the learner fully immersed in the environment. In the final analysis, the review exposed a growing trend of research in EVRGs studies since 2012 to 2018, while also revealing the application of VR in the sciences, healthcare and technology education spheres having the most significant attention. The mainstream education and arts (such as languages) gained the least interest of educators using VR. This scenario offers the opportunity for further research in the educational contexts with emphasis on the arts and humanities disciplines. It is therefore pertinent that through this systematic review, technology-mediated learning will be enhanced when there is a clear understanding of the trends concerning its application in the different domains of learning.
Availability of data and materials
Not applicable.
Abbreviations
Information and Communication Technology
Virtual Reality
Augmented Reality
Mixed Reality
Educational Virtual Reality Games
Role Playing Game
Two-Dimension
Three-Dimension
Personal Computer
Natural User Interface
Head Mounted Device
University of Eastern Finland
Microsoft Excel
Association for Computing Machinery
Institute of Electrical and Electronics Engineers
Aggarwal, R., Black, S. A., Hance, J. R., Darzi, A., & Cheshire, N. J. W. (2006). Virtual reality simulation training can improve inexperienced surgeons’ endovascular skills. European Journal of Vascular and Endovascular Surgery , 31 (6), 588–593.
Article Google Scholar
Alazba, A., Al-Khalifa, H., & AlSobayel, H. (2018). A proposed game for promoting physical activities among people with low back pain using virtual reality. In Proc. 11th PErvasive technologies related to assistive environments conference (PETRA '18) , (pp. 141–144). New York: ACM. https://doi.org/10.1145/3197768.3197780 .
Chapter Google Scholar
Alexander, B., Ashford-Rowe, K., Barajas-Muphy, N., Dobbin, G., Knott, J., & Mccormack, M. (2019). Educase horizon report . Louisville: EDUCAUSE, Higher Education Edition https://library.educause.edu/resources/2019/4/2019-horizon-report .
Google Scholar
Alrehaili, E. A. (2018). A Virtual reality role-playing serious game for experiential learning . (Master thesis), University of Ottawa. https://doi.org/10.20381/ruor-22159 .
Book Google Scholar
Amal, A., Hend, A., & Hana, A. (2018). A proposed game for promoting physical activities among people with low back pain using virtual reality. In Proceedings of the 11th PErvasive technologies related to assistive environments conference (PETRA '18) . New York: ACM.
Bekele, M. K., Pierdicca, R., Frontoni, E., Malinverni, E. S., & Gain, J. (2018). A survey of augmented, virtual, and mixed reality for cultural heritage. Journal on Computing and Cultural Heritage (JOCCH) , 11 (2), 7.
Bernardes, M., Barros, F., Simoes, M., & Castelo-Brancon, M. (2015). A serious game with virtual reality for travel training with autism Spectrum disorder. In International conference on virtual rehabilitation (ICVR) , (pp. 127–128). Valencia: IEEE. https://doi.org/10.1109/ICVR.2015.7358609 .
Boboc, A. L., Orzan, G., Stoica, I., & Niculescu-Ciocan, C. (2018). Gamification and game-based learning– A solution for Romanian education system. The International Scientific Conference eLearning and Software for Education. , 1 , 242–248.
Bouali, N., Nygren, E., Oyelere, S. S., Suhonen, J., & Cavalli-Sforza, V. (2019). A VR game to introduce OOP concepts. In 19th Koli calling international conference on computing education research (Koli calling ‘19) . New York: ACM.
Bradshaw, T. (2016). Virtual reality: four ways it could change your world. https://www.ft.com/content/0f7d7ecc-db47-11e5-a72f-1e7744c66818.html .
Bryan, S. J., Campbell, A., & Mangina, E. (2018). An AR/VR Educational Game. In IEEE games, entertainment, media conference (GEM) , (pp. 1–9). Galway. https://doi.org/10.1109/GEM.2018.8516456 .
Byl, B., Süncksen, B. M., & Teistler, M. (2018). A serious virtual reality game to train spatial cognition for medical ultrasound imaging. In IEEE 6th international conference on serious games and applications for health (SeGAH) , (pp. 1–4). https://doi.org/10.1109/SeGAH.2018.8401365 .
Calvi, L., Santos, C. P., Relouw, J., Endrovski, B., Rothwell, C., Sarà, A., … Pantaleo, U. (2017). A VR game to teach underwater sustainability while diving. In Sustainable internet and ICT for sustainability (SustainIT’ 2017) , (pp. 1–4). https://doi.org/10.23919/SustainIT.2017.8379812 .
Carvalheiro, C., Nóbrega, R., da Silva, H., & Rodrigues, R. (2016). User redirection and direct haptics in virtual environments. In Proceedings of the 24th ACM international conference on multimedia , (vol. 5, pp. 1146–1155).
Chin, C. S., Kamsani, N. B., Zhong, X., Cui, R., & Yang, C. (2018). Unity3D serious game engine for high fidelity virtual reality training of remotely-Operated Vehicle Pilot. In Proc. 10th international conference on Modelling, identification and control (ICMIC), Guiyang , (pp. 1–6). https://doi.org/10.1109/ICMIC.2018.8529900 .
Chittaro, L., & Buttussi, F. (2015). Assessing knowledge retention of an immersive serious game vs. a traditional education method in aviation safety. In IEEE Transactions on Visualization and Computer Graphics. 21(4), 529–538. doi: https://doi.org/10.1109/TVCG.2015.2391853 .
Chiu, F. (2017). Virtual reality for learning languages based on mobile devices. In 16th international conference on information technology based higher education and training (ITHET) , (pp. 1–3). https://doi.org/10.1109/ITHET.2017.8067813 .
Christou, C. (2010). Virtual Reality in Education. In Affective, interactive and cognitive methods for e-learning design: creating an optimal education experience , (pp. 228–243).
Cohen, C. A., & Hegarty, M. (2014). Visualizing cross sections: Training spatial thinking using interactive animations and virtual objects. Learning and Individual Differences. , 33 , 63–71.
Diez, H. V., Andoni, S. G., Aitor, M., & Oyarzun, D. (2016). Virtual training of fire wardens through immersive 3D environments. In Proceedings of the 21st international conference on Web3D technology (Web3D '16) , (pp. 43–50). New York: ACM. https://doi.org/10.1145/2945292.2945296 .
Dinis, F. M., Guimarães, A. S., Carvalho, B. R., & Martins, J. P. P. (2017). Development of virtual reality game-based interfaces for civil engineering education. In IEEE global engineering education conference (EDUCON) , (pp. 1195–1202). https://doi.org/10.1109/EDUCON.2017.7943000 .
Duffy, T. M., & Jonassen, D. H. (1992). Constructivism and the designing of learning Environments: Technology of instruction: A conversation. Hillsdale N.J.: Lawrence Erlbaum. Computer and System Sciences , 105 , 87–108. https://doi.org/10.1007/978-3-642-78069-1 .
Finkelstein, S., Nickel, A., Lipps, Z., Barnes, T., Wartell, Z., & Suma, E. A. (2011). Astrojumper: motivating exercise with an immersive virtual reality Exergame. Presence Teleoperators and Virtual Environments. , 20 (1), 78–92.
Greenwald, S. W., Kulik, A., Kunert, A., Beck, S., Fröhlich, B., & Cobb, S. (2017). Technology and Applications for Collaborative Learning in Virtual Reality. In Smith. Making a Difference: Prioritizing Equity and Access in CSCL. In 12th international conference on computer supported collaborative learning (CSCL) , (vol. 2).
Gyutae, H., Hojun, L., Sangho, L., Jaekwang, C., & Shiho, K. (2016). A VR serious game for fire evacuation drill with synchronized tele-collaboration among users. In 22nd ACM conference on virtual reality software and technology (VRST '16) , (pp. 301–302). New York: ACM. https://doi.org/10.1145/2993369.2996306 .
Hafsia, M., Monacelli, E., & Martin, H. (2018). Virtual reality simulator for construction workers. In Proc. virtual reality international conference - Laval virtual (VRIC '18) . https://doi.org/10.1145/3234253.3234298 .
Hentsch, C. (2018, February, 9). Virtual Reality in Education: How VR can be Beneficial to the Classroom. https://edtechtimes.com/2018/02/09 .
Heuven, R., Kinast, B., Thomas, A., Tabak, M., Cabrita, M., Schüler, T., & Hörst, D. (2017). Dinner is ready!: Virtual Reality Assisted Training for Chronic Pain Rehabilitation. In ACM SIGCHI Annual Symposium on Computer-Human Interaction in Play (CHI PLAY) , (vol. 17, pp. 283–289). CHI PLAY’.
Hwang, G. J., Wu, P. H., Chen, C. C., & Tu, N. T. (2016). Effects of an augmented reality-based educational game on students’ learning achievements and attitudes in real-world observations. Interactive Learning Environments. , 24 (8), 1895–1906.
Kaliisa, R., Edward, P., & Julia, M. (2019). Mobile learning in higher education: A comparative analysis of developed and developing country contexts. British Journal of Educational Technology. , 50 (2), 546–561.
Kitchenham, B., & Charters, S. (2007). Guidelines for performing systematic literature reviews in software engineering. Engineering. , 45 (4), 1051.
Knote, A., Edenhofer, S., & Mammen, S. V. (2016). Neozoa: An immersive, interactive sandbox for the study of competing. In IEEE virtual reality workshop on K-12 Embodied Learning through Virtual & Augmented Reality (KELVAR) , (pp. 5–10). https://doi.org/10.1109/KELVAR.2016.7563675 .
Laine, T. H. (2018). Mobile educational augmented reality games: A systematic literature review and two case studies. Computers. , 7 (19), 11–15.
Lee, E. A. L., & Wong, K. W. (2008). A review of using virtual reality for learning. Transactions on Edutainment , 1 , 231–241.
Lee, E. A. L., & Wong, K. W. (2014). Learning with desktop virtual reality: Low spatial ability learners are more positively affected. Computers & Education. , 79 , 49–58.
Lee, E. A. L., Wong, K. W., & Fung, C. C. (2010). How does desktop virtual reality enhance learning outcomes? A structural equation modeling approach. Computers & Education , 55 (4), 1424–1442 https// psycnet.apa.org >record.
Lele, A. (2013). Virtual reality and its military utility. Journal of Ambient Intelligent Human Computer. , 4 , 17–26.
Likitweerawong, K., & Palee, P. (2018). The virtual reality serious game for learning driving skills before taking practical test. In International Conference on Digital Arts, Media and technology (ICDAMT) , (pp. 158–161). https://doi.org/10.1109/ICDAMT.2018.8376515 .
Linden, A., & Fenn, J. (2003). Understanding Gartner’s hype cycles. In Strategic Analysis Report Gartner, Inc. , (pp. 20–1971).
Liu, W., Cheok, A. D., Mei-Ling, C. L., & Theng, Y. L. (2007). Mixed reality classroom - learning from entertainment. ACM. , 1 (1), 65–72.
Lovreglio, R., Gonzalez, V., Feng, Z., & Sacks, R. (2018). Prototyping virtual reality serious games for building earthquake preparedness: The Auckland City Hospital case study. Advanced Engineering Informatics, 38, 670–682.
Mandal, S. (2013). Brief introduction of virtual reality & its challenges. International Journal of Scientific & Engineering Research. , 4 (4), 304.
Mihajlovic, Z., Popovic, S., & Brkic, K. (2018). A system for head – Neck rehabilitation exercises based on serious gaming virtual reality. Multimed Tools and Applications. , 77 (15), 19113–19137.
Moher, D., Liberati, A., Tetzlaff, J., & Altman, D. G. (2009). The PRISMA Group (2009) preferred reporting items for systematic reviews and meta-analyses. PLoS Med. , 6 (7):e1000097. https://doi.org/10.1371/journal.pmed.1000097 .
Moldoveanu, A. D. (2017). Virtual environments for training visually impaired for a sensory substitution device. In Zooming Innovation in Consumer Electronics International Conference (ZINC) , (pp. 26–29). https://doi.org/10.1109/ZINC.2017.7968654 .
Nicas, J. (2018). What does virtual reality do to your body and mind? Wall Str J. http://www.wsj.com/articles/what-does-virtual-reality-do-to-your-body-and-mind-1451858778 .
Oyelere, S. S., Suhonen, J., & Laine, T. H. (2017). Integrating Parson’s programming puzzles into a game-based mobile learning application. In Proc. Koli calling international conference on computing education research , (pp. 158–162).
Oyelere, S. S., Suhonen, J., Wajiga, G. M., & Sutinen, E. (2018). Design, development, and evaluation of a mobile learning application for computing education. Education and Information Technologies, Springer. , 23 (1), 467–495.
Pavlidis, G. P., & Markantonatou, S. (2018). Playful education and innovative gamified learning approaches. In Handbook of Research on Educational Design and Cloud Computing in Modern Classroom Settings , (pp. 321–341).
Pirker, J., Lesjak, I., & Guetl, C. (2017). Maroon VR: A Room-Scale Physics Laboratory Experience. In IEEE 17th International Conference on Advanced Learning Technologies (ICALT) , (pp. 482–484). https://doi.org/10.1109/ICALT.2017.92 .
Puttawong, N., Visoottiviseth, V., & Haga, J. (2017). VRFiWall virtual reality edutainment for firewall security concepts . In 2nd International Conference on Information Technology (INCIT) , (pp. 1–6). https://doi.org/10.1109/INCIT.2017.8257864 .
Roberto, A., Angela, C., Armando, F., Delfina, M., Donato, P., & Mirta, R. (2017). A framework to design, develop, and evaluate immersive and collaborative serious games in cultural heritage. Journal on Computing and Cultural Heritage , 11 (1). https://doi.org/10.1145/3064644 .
Roettl, J., & Terlutter, R. (2018). The same video game in 2D, 3D or virtual reality–how does technology impact game evaluation and brand placements. PLoS One , 13 (7), e0200724.
Sampaio, A. Z., Ferreira, M. M., Rosário, D. P., & Martins, O. P. (2010). 3D and VR models in civil engineering education: Construction, rehabilitation and maintenance. Automation in Construction. , 19 (7), 819–828.
Sárkány, A. (2016). Maintain and improve mental health by smart virtual reality serious games. In Pervasive computing paradigms for mental health , (vol. 604). Cham: MindCare. Communications in Computer and Information Science, Springer.
Shahab, M. (2017). Social virtual reality robot (V2R): A novel concept for education and rehabilitation of children with autism. In 5th RSI International Conference on Robotics and Mechatronics (ICRoM) , (pp. 82–87). https://doi.org/10.1109/ICRoM.2017.8466148 .
Sharkawi, K. H., Ujang, M. U., & Abdul-Rahman, A. (2008). 3D navigation system for virtual reality based on 3D game engine. In The international archives of the photogrammetry, remote sensing and spatial information sciences, 37(PART B4) .
Shin, D. H. (2017). The role of affordance in the experience of virtual reality learning. Telematics & Informatics. , 34 (8), 1826–1836.
Snowdon, C. M., & Oikonomou, A. (2018). Analysing the educational benefits of 3D virtual learning environments. In European conference on e-learning (513-XVIII) . Kidmore End: Academic Conferences International Limited.
Süncksen, M., Bendig, H., Teistler, M., Wagner, M., Bott, O. J., & Dresing, K. (2018). Gamification and virtual reality for teaching mobile x-ray imaging. In IEEE 6th international conference on Serious Games and Applications for Health (SeGAH) , (vol. 7). https://doi.org/10.1109/SeGAH.2018.8401364 .
Szczurowski, K., & Smith, M. (2018). “Woodlands” - a virtual reality serious game supporting learning of practical road safety skills. In IEEE Games, Entertainment, Media conference (GEM) , (pp. 1–9). https://doi.org/10.1109/GEM.2018.8516493 .
Tabak, M., Miriam, C., Thomas, S., David, H., René, H., & Benjamin, K. (2017). " Dinner is ready!": Virtual Reality Assisted Training for Chronic Pain Rehabilitation. In In extended abstracts publication of the annual symposium on computer-human interaction in play (CHI PLAY '17 extended abstracts) , (pp. 283–289). ACM.
Triberti, S., Villani, D., & Riva, G. (2016). Unconscious goal pursuit primes attitudes towards technology usage: A virtual reality experiment. Computers in Human Behavior. , 64 , 163–172.
Trombetta, M., Henrique, P. P. B., Brum, M. R., Colussi, E. L., Marchi, A. C. B., & Rieder, R. (2017). Motion rehab AVE 3D: A VR-based exergame for post-stroke rehabilitation. Computer Methods and Programs in Biomedicine. , 151 , 15–20.
Tsoy, D., Sneath, P., Rempel, J., Huang, S., Bodnariuc, N., & Mercuri, M. (2019). Creating GridlockED: A serious game for teaching about multipatient environments. Academic Medicine. , 94 (1), 66–70.
Tussyadiah, L. P., Wang, D., Jung, T. H., & Dieck, M. C. (2018). Virtual reality, presence, and attitude change: Empirical evidence from tourism. Tourism Management. , 66 , 140–154.
Vesisenaho, M., Juntunen, M., Häkkinen, P., Pöysä-Tarhonen, J., Fagerlund, J., & Miakush, I. (2019). Virtual reality in education: Focus on the role of emotions and physiological reactivity. Journal of Virtual Worlds Research. , 12 (1). https://doi.org/10.4101/jvwr.v12i1.7329. .
Virvou, M., & Katsionis, G. (2008). On the usability and likeability of virtual reality games for education: The case of VR-ENGAGE. Computers & Education , 50 (1):154–178.
Visoottiviseth, V., Phungphat, A., Puttawong, N., Chantaraumporn, P., & Haga, J. (2018). Lord of Secure: the Virtual Reality Game for Educating Network Security. In Seventh ICT International Student Project Conference (ICT-ISPC) , (pp. 1–6). https://doi.org/10.1109/ICT-ISPC.2018.8523947 .
Voinea, G. D., Girbacia, F., Postelnicu, C. C., & Marto, A. (2018). Exploring Cultural Heritage Using Augmented Reality Through Google’s Project Tango and ARCore. In International conference on VR Technologies in Cultural Heritage , (pp. 93–106).
Vourvopoulos, A., Faria, A. L., Ponnam, K., & Badia, S. B. (2014). RehabCity: design and validation of a cognitive assessment and rehabilitation tool through gamified simulations of activities of daily living. In Proc. 11th conference on advances in computer entertainment technology (ACE '14), Funchal, Portugal . https://doi.org/10.1145/2663806.2663852 .
Xenos, M., Maratou, V., Ntokas, I., Mettouris, C., & Papadopoulos, G. A. (2017). Game-based learning using a 3D virtual world in computer engineering education. In IEEE global Engineering Education Conference (EDUCON) .
Zielke, M. A. (2017). Developing Virtual Patients with VR/AR for a natural user interface in medical teaching. In IEEE 5th international conference on Serious Games and Applications for Health (SeGAH) , (pp. 1–8). https://doi.org/10.1109/SeGAH.2017.7939285 .
Zikky, M., Fathoni, K., & Firdaus, M. (2018). Interactive distance media learning collaborative based on virtual reality with solar system subject. In 19th IEEE/ACIS international conference on software engineering, Artificial Intelligence, Networking and Parallel/Distributed Computing (SNPD) , (pp. 4–9). https://doi.org/10.1109/SNPD.2018.8441031 .
Zyda, M. (2005). From visual simulation to virtual reality to games. IEEE Computer Society. 37 (9), 25–32. https://doi.org/10.1109/MC.2005.297 .
Download references
Acknowledgements
Author information, authors and affiliations.
University of Eastern Finland, School of Computing, Joensuu, Finland
Solomon Sunday Oyelere & Nacir Bouali
Faculty of Electrical Engineering, Mathematics and Computer Science, University of Twente, Enschede, NB, 7522, The Netherlands
Nacir Bouali
Department of Education, University of Oslo, Oslo, Norway
Rogers Kaliisa
University of the Witwatersrand, Johannesburg, South Africa
George Obaido
Usmanu Danfodiyo University, Sokoto, Nigeria
Abdullahi Abubakar Yunusa
Crown Hill University, Eiyekorin, Ilorin, Kwara State, Nigeria
Ebunayo R. Jimoh
You can also search for this author in PubMed Google Scholar
Contributions
The first author made substantial contributions to the conception and design of the work. The author was involved in the acquisition, analysis, and interpretation of Google scholar data for the work. The author was involved in the drafting the methodology, discussion and conclusion part of the work and revising it critically for important intellectual content. The author was responsible for making final approval of the manuscript to be submitted for review. The second author made substantial contributions to the design of the literature review process. The author was involved in the acquisition of data from IEEE database, analysis, and interpretation of data for the work. The author was involved in the drafting of the result and discussion section of the work and revising it critically for important intellectual content. The third author made substantial contributions and participated in data searching in ScienceDirect database, collection and analysis, writing and reviewing the article. The author was involved in the drafting of some aspect of the literature review section of the work and revising it critically for important intellectual content. The fourth author made substantial contributions to the design of the literature review process. The author was involved in the acquisition of data from Google scholar database, analysis, and interpretation of data for the work. The author was involved in the drafting of the result and discussion section of the work, proofreading, and revising it critically for important intellectual content. The fifth author made substantial contributions and reviewed articles related to ACM database within the scope of the systematic review. The author was involved in drafting some aspects of the literature review section. The author was also responsible for analysis, and interpretation of data for the work and edited some portions of the manuscript and drafted the conclusion as well as the abstract. The sixth author made substantial contributions and participated in data searching in IEEE database, collection and analysis, and reviewing the article. The author was involved in the drafting of some aspects of the literature review section of the work and revising it critically for important intellectual content. General note: It is important to mention here that the manuscript went through circles of reading, proofreading and editing by all the authors. The authors' read and approved the final manuscript.
Corresponding author
Correspondence to Solomon Sunday Oyelere .
Ethics declarations
Competing interests.
No competing interests.
Additional information
Publisher’s note.
Springer Nature remains neutral with regard to jurisdictional claims in published maps and institutional affiliations.
Rights and permissions
Open Access This article is licensed under a Creative Commons Attribution 4.0 International License, which permits use, sharing, adaptation, distribution and reproduction in any medium or format, as long as you give appropriate credit to the original author(s) and the source, provide a link to the Creative Commons licence, and indicate if changes were made. The images or other third party material in this article are included in the article's Creative Commons licence, unless indicated otherwise in a credit line to the material. If material is not included in the article's Creative Commons licence and your intended use is not permitted by statutory regulation or exceeds the permitted use, you will need to obtain permission directly from the copyright holder. To view a copy of this licence, visit http://creativecommons.org/licenses/by/4.0/ .
Reprints and permissions
About this article
Cite this article.
Oyelere, S.S., Bouali, N., Kaliisa, R. et al. Exploring the trends of educational virtual reality games: a systematic review of empirical studies. Smart Learn. Environ. 7 , 31 (2020). https://doi.org/10.1186/s40561-020-00142-7
Download citation
Received : 29 July 2020
Accepted : 06 October 2020
Published : 19 October 2020
DOI : https://doi.org/10.1186/s40561-020-00142-7
Share this article
Anyone you share the following link with will be able to read this content:
Sorry, a shareable link is not currently available for this article.
Provided by the Springer Nature SharedIt content-sharing initiative
- Educational games
- Virtual reality
- Educational virtual reality games
- Systematic review

An official website of the United States government
The .gov means it’s official. Federal government websites often end in .gov or .mil. Before sharing sensitive information, make sure you’re on a federal government site.
The site is secure. The https:// ensures that you are connecting to the official website and that any information you provide is encrypted and transmitted securely.
- Publications
- Account settings
Preview improvements coming to the PMC website in October 2024. Learn More or Try it out now .
- Advanced Search
- Journal List
- Int J Environ Res Public Health

How Virtual Reality Technology Has Changed Our Lives: An Overview of the Current and Potential Applications and Limitations
Associated data.
No new data were created or analyzed in this study. Data sharing is not applicable to this article.
Despite virtual reality (VR) being initially marketed toward gaming, there are many potential and existing VR applications in various sectors and fields, including education, training, simulations, and even in exercise and healthcare. Unfortunately, there is still a lack of general understanding of the strengths and limitations of VR as a technology in various application domains. Therefore, the aim of this literature review is to contribute to the library of literature concerning VR technology, its applications in everyday use, and some of its existing drawbacks. Key VR applications were discussed in terms of how they are currently utilized or can be utilized in the future, spanning fields such as medicine, engineering, education, and entertainment. The main benefits of VR are expressed through the text, followed by a discussion of some of the main limitations of current VR technologies and how they can be mitigated or improved. Overall, this literature review shows how virtual reality technology has the potential to be a greatly beneficial tool in a multitude of applications and a wide variety of fields. VR as a technology is still in its early stages, but more people are becoming interested in it and are optimistic about seeing what kind of changes VR can make in their everyday lives. With how rapidly modern society has adapted to personal computers and smartphones, VR has the opportunity to become the next big technological turning point that will eventually become commonplace in most households.
1. Introduction
This literature review aims to contribute to the library of literature on the applications of virtual reality (VR), how they are currently used and can be used in the future, and some of the strengths and difficulties that come with using VR.
Virtual reality (VR) refers to a computer-generated, three-dimensional virtual environment that users can interact with, typically accessed via a computer that is capable of projecting 3D information via a display, which can be isolated screens or a wearable display, e.g., a head-mounted display (HMD), along with user identification sensors [ 1 ]. VR can mainly be divided into two categories: non-immersive, and immersive [ 2 ]. Non-immersive VR utilizes a combination of screens surrounding the user to present virtual information [ 3 ]. A typical example of this is driving or flight simulations in which the user sits in a chair with multiple screens around them, giving them the feeling of being in the cockpit or driver’s seat without being fully immersed. Immersive VR refers to using a wearable display, e.g., HMD, to track a user’s movement and present the VR information based on the position of users [ 4 ], which allows them to experience 360 degrees of the virtual environment. This immersive experience is what most people think of when it comes to VR and is one of the most marketable aspects of VR technology. In between immersive and non-immersive VR, there is also augmented reality (AR). AR makes use of computer-generated imagery that is overlayed on physical elements in the real world, which can be found in many applications, such as stores providing a virtual fitting application for people to “try on” clothes. Mixed reality (XR) represents the spectrum between the physical and digital worlds, combining AR and VR to allow users to both immerse themselves in a virtual world while also being somewhat grounded in reality.
The concept of VR was first introduced in the 1960s, with Morton’s creation of the Telesphere Mask and the Sensorama [ 5 ]. The original technologies served the purpose of immersing the user in the video display around them, making them feel like they are a part of the video. The Ultimate display was an idea developed by Ivan Sutherland [ 6 ], operating on a similar concept of allowing the user to feel immersed in a computer-generated environment using multiple input and output devices [ 7 , 8 ]. Following the creation of the Sensorama and the idea of the Ultimate display in the 1960s, the next large boom in VR technology development occurred in the early 2010s. During this period of time, VR was still considered a gimmick—it was expensive and was not considered a technology that would ever become popular with the general public. This, however, started to shift in 2012, when Palmer Luckey debuted his prototype for the first Oculus [ 9 ]. In 2014, Facebook acquired Oculus after seeing the interest it garnered, leading to a significant increase in the popularity of VR devices for home use. Since then, VR has grown to become more popular and accessible to the everyday consumer, with more VR headsets available on the market, such as the HTC Vive, Samsung VR, Oculus, Google Cardboard, and more.
Despite VR being initially marketed toward gaming, there are many potential and existing VR applications in various sectors and fields, including education, training, simulations, and even in exercise and healthcare. Unfortunately, there is still a lack of general understanding of the strengths and limitations of VR as a technology in various application domains. Some of the largest issues with current VR technology are hard to overcome and can span from technical to financial and health issues. Technological limitations regarding users feeling uncomfortable or ill while using a VR headset, the inaccessibility of this technology to most people due to the high price of the associated hardware, and the lack of technical standardization are all current issues that the tech industry is hoping to overcome with research and future improvements.
Overall, this literature review serves the purpose of covering how different types of VR applications can be utilized, as well as providing information on the advantages and drawbacks of using VR technology in various application domains.
In order to present a reliable literature review, an extensive search was performed using common journal search engines/websites, e.g., Google Scholar, JSTOR, MDPI, ResearchGate, PubMed, and Science Direct, which includes peer-reviewed studies and articles. Keywords and phrases used in searching for sources include a combination of “VR” or “virtual reality” with “Education”, “Simulation,” “Games”, “Virtual”, “Immersive”, “Non-immersive”, “Training”, “Application”, “Manufacturing”, “Industrial”, “Medical”, “Healthcare”, and “Entertainment”. The variety in keywords helped yield different results for VR not only as a technology but also in major use cases where it has already been utilized for different industries and fields. The gathered papers and articles were then reviewed to further select representative and up-to-date evidence.
Papers were selected with the goal of providing sufficient coverage of the topic by presenting an overarching summary rather than an exhaustive review of every type of application within VR. Having a large variety of papers does not guarantee that every particular use case of VR is covered, but it does provide a wide breadth of use cases of VR that are currently applied, as well as opportunity spaces for VR applications in the future. As shown in Figure 1 , 145 papers were initially collected, but only 77 were thoroughly reviewed to provide enough coverage without unnecessary advanced technical details. Five additional papers and articles were added after review to accommodate additional information, resulting in a total of 82 sources used for the final literature review.

General structure of the paper selection and literature review.
Included papers were those that clearly presented a specific VR application, those that showed clear negative or positive outcomes of VR usage, or papers that provided relevant background information on a specific VR technology. Exclusion criteria included disregarding papers that had an overt focus on VR hardware components, excluding studies that may have mentioned VR without it being the focus, and rejecting papers that became repetitive after utilizing other papers on similar topics. The following sections provide detailed reviews based on various VR applications and domains.
3. Reviews of VR Technology Applications
The technological applications of VR have advanced to a point where they can be applied to an extensive range of fields and industries outside of just gaming or entertainment. Many have started to take advantage of VR in performing tasks that are hard to practice due to limited resources or the inherent risks and dangers associated with said tasks that can sometimes lead to catastrophic consequences. The greatest strength of VR is that it opens up opportunities for people to practice these tasks in a safe capacity while also being immersed enough for it to feel realistic and transferable to the real world and depict almost any situation accurately [ 10 ]. This section covers some of the main categories of VR applications and provides examples of how these applications are applied or can be applied to different use cases across various fields.
One of the most widely used and largely applicable applications of VR is the simulation aspect, which can be uniquely created and customized to suit users’ needs. There are two main types of simulations: immersive and non-immersive. As mentioned above, non-immersive VR simulations usually include multiple screens and some type of platform or apparatus that mimics the activities or tasks in reality [ 3 ]. Immersive VR simulations differ in terms of using HMDs in place of screens and can either utilize a control platform or apparatus such as the ones used in non-immersive simulations [ 11 ] or can instead be fully contained within a virtual setup and require no external setups or platforms. Whether users opt for immersive or non-immersive VR simulations, there is no significant difference in the performance, and the results appear to be very similar in fulfilling the simulation’s purpose [ 12 ]. There is, however, a slight advantage to using immersive VR simulations with HMDs, as they are capable of fully immersing the user in the simulated environment and giving them a more thorough experience [ 13 ].
3.1. Industrial Simulation Applications
VR simulations have many applications that can span from training simulation to prototyping, designing, and testing tools and objects. Some commonly used VR simulations in the industrial domain include driving simulators, flight simulators for pilots, and combat simulators for military personnel, all of which provide training to users in highly dangerous circumstances without putting them at risk during the training process [ 14 ]. Among the many use cases, two typical simulation applications are further discussed in the following sections.
3.1.1. Driving Simulations
One major use of VR simulations is driving simulations for both driving training and within the automotive industry; VR provides the ability to create driving simulations in which users can be placed in risky driving scenarios without real danger [ 15 ]. Driving simulators can be useful in multiple capacities, such as observing driving behavior to collect data or training inexperienced drivers in a low-stress environment.
VR driving simulations can be used to train young or novice drivers and help them understand their mistakes or point out some bad driving habits they need to adjust. Within a simulation, drivers can be placed in a virtual vehicle within an environment resembling a cityscape, with their behaviors and actions observed and recorded to later analyze for any issues or mistakes or to see if the drivers made the correct decisions in a given scenario [ 16 ]. After conducting the simulation, drivers can be informed of their mistakes and receive feedback about how to improve their behaviors in an actual driving situation. These driving simulations can also be beneficial in training young drivers with neurodevelopmental disorders such as autism spectrum disorder (ASD) [ 17 ], who may otherwise have difficulties learning in an uncontrolled environment.
Another application of VR driving simulations is the ability to collect real-time data on how users react to different scenarios as drivers on the road in a simulated environment. This data can be used in multiple capacities, such as designing better safety features in a vehicle, providing a better user experience for drivers, developing training modules for drivers, and for use in autonomous vehicle (AV) research and development. AVs have been an emerging field of technology that will continue to develop and advance, with VR simulations continuously providing opportunities for safe and efficient data collection and user testing [ 18 ]. One common issue in the field is developing trust between users and autonomous vehicles and understanding how to mitigate the distrust most people have in this technology [ 19 ]. It is important to ensure users have a certain level of trust in an AV so as to ensure drivers take over when appropriate. Accordingly, putting users in a VR driving simulation in which they interact with an autonomous vehicle virtually can yield substantial amounts of data on how users behave within that environment while also ensuring that users feel safe in the process and can become accustomed to being in an AV [ 20 ].
3.1.2. Product Design and Prototyping
One application of VR that can be useful is the ability to look at 3D models in a virtual space in a way that is difficult to visualize via a screen. Prototypes or preliminary designs for products can be modeled and shown in a virtual environment for test and evaluation purposes [ 21 ]. One significant advantage of showing these models in VR is presenting a virtual prototype or part without spending a lot of time, money, effort, or material on building the prototype in real life. Through simulations, VR can also show how the product would react under different conditions. Simulations can be run in VR to show the effect of different interactions between the prototype and surrounding subjects [ 22 ]. This can help the prototype designers determine if any areas of the prototype need to be improved based on the simulated interaction results. The ability to see the product in a virtual environment can also provide the ability to make changes to VR design for a quick turnaround and faster results, which could increase the speed of prototyping, reduce prototype production waste, and increase the understanding of the functions of the prototype.
3.2. Education
Educational applications of VR have not been utilized much yet, but there are many promising examples and studies of how beneficial VR can be in an educational environment. Using VR can help increase student attention by keeping them engaged with what is happening inside the VR environment [ 23 , 24 ]. Most teenage students find it challenging to pay attention in class, especially when they feel that the discussed topics are not relevant to them. When students use exciting technologies such as VR, they are more interested and engaged with what they are learning while immersed in a virtual environment [ 25 , 26 ]. VR headsets are also useful in blocking out visual and auditory distractions, creating an opportunity for the student to focus on teaching materials better. Such VR approaches open up more opportunities for teachers to interact one-on-one with students and have more useful and beneficial teacher–student interactions [ 27 ].
VR also provides the opportunity for students to construct and practice their own knowledge by being able to engage in meaningful experiences. Students are able to immersively engage in educational activities and gain a better understanding of the topic at hand [ 28 ]. VR also has the capability of transporting students to different environments, allowing them to learn and explore various concepts safely and efficiently. This can be especially useful to demonstrate environments that are impossible to visit in reality, such as underwater or space [ 29 , 30 ].
Mixed reality can be considered an extended VR application, which can be applied to real learning environments, such as exploring laboratory experiments [ 31 ]. Students can wear an HMD that shows information and instructions about the laboratory they will experience and can interact with items in reality to recreate what is simulated to them in VR. Essentially, students are still fully aware of their surroundings while also having a better visual understanding and representation of their task, which can help reduce mistakes, allow students to be more independent, and keep students interested and engaged.
With the start of the COVID-19 pandemic, there has been a sudden increase in virtual learning, with many classes being held via online meeting platforms and others being fully asynchronous. VR offers a new, unique approach to asynchronous learning; VR can create a learning environment in which a student can participate in lectures and ask questions to virtual instructors with pre-generated answers [ 32 ]. It is particularly important for students to feel immersed in the virtual environment in order to keep them engaged [ 33 ]. Virtual environments can be created to look just like real-life classrooms where students can walk around and work with other students on assignments [ 34 ]. The issue with asynchronous classroom experiences is that not all of a student’s questions will necessarily be answered; information will be limited to what is currently updated within the virtual experience. Thus, VR-based virtual education does provide a better experience to students than watching videos online, but it cannot replace the experience of being in a classroom with teachers who can directly engage with students.
With VR technology further advancing, VR could also be used for live, synchronous classes where students can engage with classmates and teachers from the comfort of their homes in real time. This would have been especially beneficial when schools were closed due to the pandemic, but it can also provide a way for students to attend classes while experiencing health difficulties, traveling, or living in other countries, etc. Even though live classes have not yet really been held using VR, such applications can be developed in the future, especially with some of the current development being made in both asynchronous learning and social interaction.
3.3. Public Health
Another domain in which VR has been utilized is within public health and wellness. Due to the immersive nature of VR, it can be used to simulate experiences that can directly impact people’s health. Some examples include providing immersive training simulations to medical personnel, offering a new method of exercise or meditation, and presenting therapists with opportunities to better help and understand their patients.
3.3.1. Medical Training
VR simulations provide the opportunity for medical professionals to practice procedures before operating on a patient, which has proven to help provide patients with better outcomes more consistently and reduce the incidence of mistakes. Preparation and practice in VR help improve patient outcomes because medical personnel are better prepared for each patient’s unique circumstances before operating [ 35 , 36 ].
In terms of learning how to perform procedures, medical students can train in an interactive virtual environment that can be programmed with different scenarios, which allows a student to experience real-life scenarios with virtual patients [ 37 ]. The virtual environment can be programmed in a multitude of diverse ways so the student can be prepared and better accustomed to different types of scenarios they may face with future patients. The simulation can be programmed so that a video can be played, showing how to effectively use a tool or object when the user looks at it [ 38 ]. The simulation can also provide hints or step-by-step instructions to students so they know how to perform the surgery properly. All these practices are much more hands-on than reading a textbook and more realistic than practicing on mannequins with minimal risks to a real patient, which makes VR a perfect tool to assist student learning.
Medical students are not the only ones who can benefit from VR simulations; seasoned medical professionals and surgeons can also benefit from this technology. Patient-specific virtual reality simulations (PSVR) are a technology that allows doctors to practice actual upcoming operations in VR [ 39 ]. This technology allows surgeons to practice customized procedures to match their patients’ specific needs and circumstances. A patient’s medical history and physical attributes can be created in the simulation and programmed with the most likely outcomes. When a surgeon performs a task or action in the simulation, the appropriate or most likely reaction can be programmed to simulate what would occur in real life under the same circumstance. This provides an opportunity for surgeons to plan out their surgery beforehand in a virtual environment, allowing them to be better prepared and more confident in their plan for the surgery ahead [ 40 ].
3.3.2. Exergaming, Fitness and Sports
With the initial focus of VR being on gaming, developers saw an opportunity for the emergence of a genre of games called exergames, in which users participate in physical activities to achieve the goals of the game. “The core concept of exergaming rests on the idea of using vigorous body activity as the input for interacting with engaging digital game content with the hope of supplanting the sedentary activity that typifies traditional game interaction that relies on keyboards, gamepads, and joysticks” [ 41 ]. VR games tend to fall under the category of exergames by requiring the user to stand up and move around in order to interact with the environment. Games such as Beat Saber (Beat Games, Prague, Czech Republic) make the user move around frequently to fulfill the game’s requirements.
Using VR as a workout tool helps gamify exercise, which can greatly assist users in staying motivated and engaged by providing them with goals to achieve during their workout. A study performed by Segura-Orti on dialysis patients shows that patients that used VR exercises instead of conventional physical activities had an increased level of physical activity compared to those who worked out using conventional methods [ 42 , 43 ]. This is probably due to the more enjoyable experience of getting exercise in game form that real life has failed to achieve with exercise apps and challenges. Some current examples include the implementation of treadmills and stationary bicycles with VR applications that allow users to physically run/cycle in place while virtually traveling through a virtual environment. These types of immersive experiences can make users’ workouts more enjoyable and can help encourage those new to fitness to start exercising from home in a new and exciting fashion.
VR technology is also being utilized in sports, where it is used to train athletes to improve their skills and can help provide them with physical therapy and rehabilitation. In terms of athletic training, VR presents a great method of perceptual-cognitive skills training [ 44 ], where users are able to experience and learn from video-based playback in an immersive environment rather than on a screen. This can be especially useful in customizing training for players in large team sports, such as football, basketball, or soccer [ 45 ]. VR allows individuals to repeatedly practice skills with lower risks of harm, which helps reduce injury. When injuries do occur in the real world, VR can be used in the rehabilitation process by allowing athletes to train from anywhere and at any time, even in the absence of a trainer or facility.
3.3.3. Therapy and Meditation
Another use of VR is in mental health therapy and meditation. The immersive nature of VR provides the flexibility to create various types of environments or experiences. Accordingly, VR can be used to experience situations that are hard to come by in real life, or that can be dangerous to go through in real life. For example, for those who suffer from post-traumatic stress disorder (PTSD), VR can be a way to experience situations that can trigger traumatic events within a safe, controlled capacity. Specific scenarios can be recreated in a virtual environment, and the patient can experience them in the presence of a therapist in order to receive help dealing with their trauma [ 46 ]. This type of therapy is similar to exposure therapy, in which patients confront what triggers them in order to slowly heal from their trauma [ 47 ].
For people who have certain disorders that may be hard to explain with words, VR can be a safe way to put people in scenarios that may trigger their disorders and observe their behaviors. Allowing a therapist to observe the situation can give them a better insight into why their patient is reacting in a certain way, which will allow them to better treat their patient [ 48 ].
Another application of VR is to use the immersive nature of the technology for meditation purposes. With the ability to experience a calm virtual environment that fully blocks distractions, VR presents a unique form of meditation that may be otherwise difficult to achieve at home. Studies on the use of VR in meditation have shown a slight increase in positive effects and a state of mindfulness in users after the meditation experience [ 49 ]. One study showed that VR meditation was more successful in reducing pre-exam anxiety in college students than watching a meditation video, where 71% of those using VR reported lower anxiety levels compared to 47% of the control group [ 50 ]. VR mediation has been shown to be useful in calming healthcare workers, especially during the COVID-19 pandemic. Virtual reality plus neurofeedback (VR + NF) meditation was shown to decrease the user’s anger, tension, depression, vigor, fatigue, and confusion [ 51 ]. Navarro-Haro et al. experienced an immersive VR mediation simulation and reported an increase in mindfulness and a reduction in negative emotional stress [ 52 ]. They were also less sad and less angry after the simulation. Mediation experts acknowledge that meditation with VR can be an immensely helpful and unique experience that is not yet fully utilized, and studies such as the one discussed here show promising results for this use of VR.
3.4. Social Interaction
VR provides the ability to transport users to a virtual environment in which they can interact with other users. This provides an opportunity to create social connections that may otherwise be hard to create or maintain. Social interaction via VR can be especially helpful for those with autism, as it provides a way for them to practice their communication skills. Users are able to participate in virtual cognition training to better improve their social skills, such as emotion recognition, social attribution, and analogical reasoning [ 53 ]. There are even programs in which young adults with high-functioning autism can participate that are designed with the purpose of increasing their social skills. These programs train users to better recognize facial expressions, body language, and emotions from a person’s voice [ 54 ]. These programs have lasting effects on the users, as they gain the ability to recognize other people’s emotions within the training that they can carry forward in their lives.
Social virtual reality also provides a new way for people to connect over long distances. Virtual spaces can be created in a VR environment and allow users to interact with each other in a realistic setting; users can have realistic avatars and talk to each other as if they were face-to-face [ 55 ]. This method of communication can be as effective as talking to another person in real life as long as the users feel immersed in the environment. When the users are immersed in the virtual environment, they have a better sense of presence, and their responses are more genuine [ 56 ]. This was especially popular during the COVID-19 pandemic when social distancing and travel restrictions made it much harder for people to see and speak with their loved ones [ 57 ]. Being able to attend events and experience activities with others via VR has provided a substitute for real-life interactions that is more realistic than merely speaking over the phone or via video chat [ 58 ].
3.5. Entertainment
The most prominent application of VR among the general public is within the sphere of entertainment, with VR offering new ways for users to experience several types of media in an immersive capacity.
One such form of media consumption within VR is watching movies, shows, or videos. VR offers new ways for users to experience visual media due to its ability to immerse users in a virtual world. VR displays are able to play 360° videos and allow the users to move around in the virtual environment, which provides the user with a more immersive experience and allows them to interact with the world as they see fit [ 59 ]. Users now have more control over what they want to pay attention to in a video and can experience videos in a whole new way.
Another application is virtual travel and tourism. Virtual tourism allows users to experience immersive tourism in simulated environments based on real landscapes or locations. This can make travel attainable to many people that would otherwise not be able to afford the time or money needed to physically visit faraway destinations. Examples of VR tourism include virtual museum visits, navigating areas using applications such as Google Street View, and virtual tours of popular destinations such as the Grand Canyon or the Great Wall of China. The concept of virtually visiting other countries or worlds has existed since the 90s [ 60 ], but there was a boost in interest recently due to travel constraints during the COVID-19 pandemic [ 61 ], with more people seeking travel experiences from the confines of their homes.
Live music is another form of entertainment that seems to be gaining traction as another large application of VR. Virtual reality has the ability to change the way people experience concerts, offering users the ability to attend and enjoy concerts from anywhere in the world. Prerecorded concerts are already available as a VR experience, with videos of the concerts filmed in 360 using omnidirectional cameras, allowing users to move their heads around and feel like they are physically present at the concert [ 62 ]. This can be an opportunity for users who do not have the ability to travel or could not get tickets to still enjoy the show. This will also allow users to see parts of the concert they could not see even if they were there due to cameras either being positioned on stage or close to the stage. The livestreaming of concerts in VR is still not technologically applicable, but it seems like the music industry is aiming to make it a reality at some point in the future with further VR development. As part of the most significant applications of VR, gaming has gained huge popularity recently, with headsets becoming more accessible and game developers investing more in the VR landscape. Many users have purchased VR headsets to play popular games such as Beat Saber , Super-Hot , and Job Simulator (Menlo Park, Prague, Czech Republic), some of the top-selling VR games. Besides designated VR games, many other games that were not initially made for VR are also being developed to include this capability and expand the options gamers have concerning their in-game experience. The rise of VR gaming popularity in recent years owes to the immersive capabilities of HMDs to immerse the users in the game environment, blocking out all external distractions [ 63 ] and giving the users a better sense of presence [ 64 ]. Players can experience the game from their point of view, which allows users to experience games in a whole new way [ 65 ].
4. Limitations and Side Effects of VR
Despite VR being a powerful and versatile tool, current VR technology has some evident limitations and drawbacks. These limitations include technological limits on what VR can do, how accessible VR is to the general public, and some of the side effects of using VR devices.
4.1. Technological Limitations
As a technology still in the earlier stages of development on a grand scale, VR has made significant leaps in evolution. Still, more substantial progress must occur before VR can be fully utilized in all possible applications and purposes.
Right now, the standardization of VR technology and presentation is still limited [ 66 ]; every developer may have their own interface specifications and functionality associated with their technology, and applications are not easily transferable between devices. The only standardization that can be observed as of now tends to be with popular games that are developed to be used across different VR platforms. It is also hard to troubleshoot bugs and receive proper support for any issues due to the lack of standardization. Hopefully, with time and progress in VR development, the technology can become more streamlined and provide better usability for users and transferability between devices. There are currently efforts to standardize VR, but these efforts are new, and the process is still in its infancy [ 67 ].
Other issues include hardware and software requirements for professional VR development, as most VR development software tends to take up a lot of data space on computers and have high-power consumption [ 68 ]. VR headsets also tend to be very heavy and can cause physical strain on users, causing headaches and pain, especially around the neck and shoulders [ 69 ]. As of now, it is not yet known what kind of detrimental effects VR use will have on users’ eyesight, but it is known that it can cause strain, especially with prolonged usage [ 70 ].
Another common issue is the lag between the user’s movements and the visual display within a VR headset [ 71 ]. A lot of the time, the headset’s tracking does not keep up properly with the user’s movements, which not only decreases their immersion but can also cause dizziness or “cybersickness,” which is explained in more detail below [ 71 , 72 ].
Cybersickness
One of the crucial issues with VR usage is VR-induced motion sickness, or “cybersickness” [ 73 , 74 ]. Cybersickness is a phenomenon where users will feel symptoms similar to motion sickness (i.e., nausea, dizziness, lightheadedness) as a result of using a VR device [ 71 ]. It is not yet known exactly why this occurs, but there are a few theories to explain this phenomenon. The most likely theory is known as the “sensory conflict theory,” which states that the excessive mismatch between the motion a user perceives visually and the lack of the corresponding movement in their body causes a conflict [ 71 , 72 , 75 ]. This happens when there is a disparity between the user’s visual system and vestibular system, which is the sensory system responsible for providing the brain with information about motion, head position, and spatial orientation [ 76 ]. Another explanation for cybersickness is the “ecological hypothesis”, which states that when people are not able to perceive or react to new dynamic situations, postural instability occurs [ 77 ].
Cybersickness does not always come with virtual experiences, but the issue can be exacerbated by several factors. Some individual factors include prolonged VR exposure; the user’s predisposition to motion sickness, fatigue, or nausea; and how adapted a user is to VR applications [ 71 , 78 ]. Cybersickness symptoms also seem to be less frequent when users are sitting instead of standing. Symptoms tend to worsen when a user is experiencing a high-speed simulation or game. Being a passive participant makes users more susceptible to symptoms than when they are in control of the simulation [ 71 , 79 , 80 ].
There are also some technical factors that can increase the likelihood of cybersickness occurring. These issues include noticeable lags (delays in the visual display can cause symptoms), position tracking errors (better head tracking reduces symptoms), and flicker in the visual display [ 71 , 72 ].
Cybersickness is one of the most uncomfortable issues that comes with VR usage, and if users continue to experience these uncomfortable symptoms, this can present a huge hindrance to the widespread development and utilization of VR applications [ 72 , 77 ].
4.2. Accessibility
As VR technology evolves, it is becoming more accessible, especially compared to its earlier stages. The cost of VR headsets on the market is still higher than most people can afford, but their current pricing is on par with most gaming consoles. Headsets such as Oculus Quest 2 cost about $300 for the base model and can be fully operated without the need for a computer, making it one of the more accessible headsets on the market. Most other headsets require using a computer that is “VR-ready”, meaning a high-end computer with a powerful graphics card that can manage VR applications. VR-ready computers tend to be more expensive than most computers, making this type of VR headset more expensive overall and out of reach for most people. This makes cost one of the larger barriers for people to get into VR as regular consumers, which is a hindrance to the growth of VR as a household technology.
VR as a field also includes augmented reality (AR) and mixed reality (XR), which are less immersive forms of virtual experiences where users still operate in the real world with a virtual overlay. AR and XR applications are more accessible to people due to their development for use on mobile devices, which are much more common with most people owning or having access to one. A common example of this type of application is AR games such as the popular Pokémon Go , which combines using a smartphone with a physical exploration of the real world [ 81 ] in search of “Pokémon” around them that can only be observed via their phones. Distances are tracked based on a user’s steps, and users can connect fitness apps to the game in order to increase rewards gained from crossing long distances. These types of games and applications can encourage people to be more physically active by gamifying the walking experience [ 82 ]. Similar smartphone games and applications can be a more accessible entry point for people interested in VR but who lack the funds to invest in an immersive headset and computer setup.
5. Conclusions
This literature review has shown how virtual reality technology has the potential to be a greatly beneficial tool in a multitude of applications and a wide variety of fields. Current applications span different domains such as engineering, education, medicine, and entertainment. With VR technology gaining popularity and traction, more VR applications can be further utilized in the future, both in improving current use cases as well as expanding to more domains. The hope is that with more VR technological breakthroughs and development, the current limitations and issues can be overcome, making long-term VR usage more realistic and accessible to more people.
Overall, VR as a technology is still in its early stages, but more people are becoming interested in it and are optimistic about seeing what kind of changes VR can make in their everyday lives. However, more and more application scenarios are under development by experts from different fields, which allows for more specific applications and development. With how rapidly modern society has adapted to personal computers and smartphones, VR has the opportunity to become the next big technological turning point that will eventually become commonplace in most households.
Funding Statement
This research received no external funding.
Author Contributions
Conceptualization, A.H. and B.J. methodology, A.H. and B.J. validation, B.J.; formal analysis, A.H.; investigation, A.H.; resources, A.H.; data curation, A.H.; writing—original draft preparation, A.H.; writing—review and editing, B.J.; visualization, A.H.; supervision, B.J. All authors have read and agreed to the published version of the manuscript.
Institutional Review Board Statement
Not applicable.
Informed Consent Statement
Data availability statement, conflicts of interest.
The authors declare no conflict of interest.
Publisher’s Note: MDPI stays neutral with regard to jurisdictional claims in published maps and institutional affiliations.
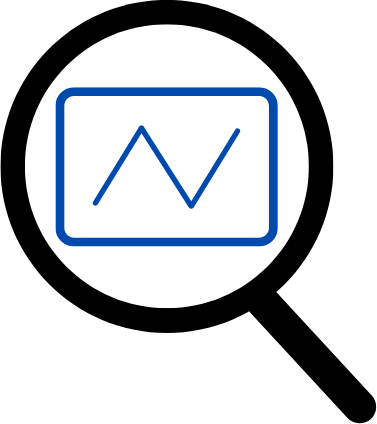
Presentations made painless
- Get Premium
107 Virtual Reality Essay Topic Ideas & Examples
Inside This Article
Virtual reality (VR) technology has become increasingly popular in recent years, offering users a fully immersive and interactive experience in a digital environment. With the rise of VR applications in various industries such as gaming, education, healthcare, and entertainment, the possibilities for exploring this technology are endless. If you are looking for inspiration for your next essay on virtual reality, here are 107 topic ideas and examples to get you started:
- The history and evolution of virtual reality technology
- The impact of VR on the gaming industry
- Virtual reality as a tool for education and training
- The use of VR in healthcare for medical simulations and therapy
- Virtual reality and its potential for treating phobias and anxiety disorders
- The ethical implications of using VR in therapy and treatment
- Virtual reality and its role in shaping the future of remote work
- The benefits of using VR for virtual meetings and conferences
- Virtual reality as a tool for creating immersive art and experiences
- The use of VR in architecture and design for virtual walkthroughs
- Virtual reality and its impact on the tourism industry
- The potential of VR for creating virtual travel experiences
- The use of VR in sports training and performance analysis
- Virtual reality and its role in enhancing the shopping experience
- The use of VR in marketing and advertising campaigns
- Virtual reality and its potential for storytelling and narrative experiences
- The impact of VR on social interactions and virtual communities
- Virtual reality and its role in preserving cultural heritage and history
- The use of VR in environmental conservation and awareness campaigns
- Virtual reality and its potential for creating virtual reality theme parks
- The benefits of using VR in disaster response and emergency training
- Virtual reality and its role in enhancing the learning experience for students
- The use of VR in virtual field trips and exploration
- Virtual reality and its impact on mental health and well-being
- The potential of VR for creating virtual reality concerts and music experiences
- The use of VR in virtual reality therapy for PTSD and trauma survivors
- Virtual reality and its role in creating virtual reality escape rooms
- The benefits of using VR in virtual reality fitness and exercise programs
- Virtual reality and its impact on the future of entertainment and media
- The use of VR in virtual reality film production and storytelling
- Virtual reality and its potential for creating virtual reality museums and exhibits
- The ethical implications of using VR in creating virtual reality experiences
- Virtual reality and its role in enhancing virtual reality sports broadcasts
- The benefits of using VR in virtual reality shopping and retail experiences
- Virtual reality and its impact on the future of virtual reality fashion and design
- The use of VR in virtual reality art installations and exhibitions
- Virtual reality and its potential for creating virtual reality music festivals
- The impact of VR on virtual reality theater and live performances
- Virtual reality and its role in enhancing virtual reality travel experiences
- The benefits of using VR in virtual reality culinary experiences
- Virtual reality and its impact on the future of virtual reality documentaries
- The use of VR in virtual reality wildlife conservation and awareness campaigns
- Virtual reality and its potential for creating virtual reality amusement parks
- The ethical implications of using VR in creating virtual reality horror experiences
- Virtual reality and its role in enhancing virtual reality fashion shows
- The benefits of using VR in virtual reality sports training and analysis
- Virtual reality and its impact on the future of virtual reality education
- The use of VR in virtual reality language learning and immersion programs
- Virtual reality and its potential for creating virtual reality historical reenactments
- The impact of VR on virtual reality meditation and mindfulness practices
- Virtual reality and its role in enhancing virtual reality team-building exercises
- The benefits of using VR in virtual reality cooking and culinary classes
- Virtual reality and its impact on the future of virtual reality wellness and self-care
- The use of VR in virtual reality gardening and nature experiences
- Virtual reality and its potential for creating virtual reality pet adoption events
- The ethical implications of using VR in creating virtual reality animal encounters
- Virtual reality and its role in enhancing virtual reality painting and art classes
- The benefits of using VR in virtual reality dance and fitness classes
- Virtual reality and its impact on the future of virtual reality social events
- The use of VR in virtual reality team-building and leadership training
- Virtual reality and its potential for creating virtual reality networking events
- The impact of VR on virtual reality educational games and simulations
- Virtual reality and its role in enhancing virtual reality storytelling and narrative experiences
- The benefits of using VR in virtual reality cultural exchange programs
- Virtual reality and its impact on the future of virtual reality language learning
- The use of VR in virtual reality cooking and culinary experiences
- Virtual reality and its potential for creating virtual reality wildlife conservation programs
- The ethical implications of using VR in creating virtual reality empathy experiences
- Virtual reality and its role in enhancing virtual reality mindfulness and meditation practices
- The benefits of using VR in virtual reality wellness and self-care programs
- Virtual reality and its impact on the future of virtual reality mental health support
- The use of VR in virtual reality pet therapy and animal encounters
- Virtual reality and its potential for creating virtual reality music therapy programs
- The impact of VR on virtual reality art therapy and creative expression
- Virtual reality and its role in enhancing virtual reality dance therapy programs
- The benefits of using VR in virtual reality drama therapy and role-playing exercises
- Virtual reality and its impact on the future of virtual reality storytelling and narrative therapy
- The use of VR in virtual reality group therapy and support groups
- Virtual reality and its potential for creating virtual reality trauma therapy programs
- The ethical implications of using VR in creating virtual reality therapy experiences
Whether you are interested in exploring the potential of virtual reality in education, healthcare, entertainment, or other industries, there are plenty of exciting topics to delve into. With these 107 virtual reality essay topic ideas and examples, you can start brainstorming your next essay on this innovative technology and its impact on society.
Want to create a presentation now?
Instantly Create A Deck
Let PitchGrade do this for me
Hassle Free
We will create your text and designs for you. Sit back and relax while we do the work.
Explore More Content
- Privacy Policy
- Terms of Service
© 2023 Pitchgrade
Using virtual reality in education
Ieee account.
- Change Username/Password
- Update Address
Purchase Details
- Payment Options
- Order History
- View Purchased Documents
Profile Information
- Communications Preferences
- Profession and Education
- Technical Interests
- US & Canada: +1 800 678 4333
- Worldwide: +1 732 981 0060
- Contact & Support
- About IEEE Xplore
- Accessibility
- Terms of Use
- Nondiscrimination Policy
- Privacy & Opting Out of Cookies
A not-for-profit organization, IEEE is the world's largest technical professional organization dedicated to advancing technology for the benefit of humanity. © Copyright 2024 IEEE - All rights reserved. Use of this web site signifies your agreement to the terms and conditions.

IMAGES
VIDEO
COMMENTS
Virtual reality in education often involves viewing or interacting with learning content using a VR headset along with any associated hardware, such as controllers that can let the user navigate and manipulate a simulated reality. VR headsets use screens, lenses and other advanced technology like sensors that are designed to wrap the viewer in ...
Introduction. VR is one of the most studied emerging innovative technologies used in several fields of education such as medical training, military training and architectural design learning (Cipresso et al., Citation 2018), VR applications have gained popularity in education research due to their positive impact on motivation, increased time on-task, memory retention and enjoyment (Kavanagh ...
The utilisation of virtual reality and virtual environments in education has a long history, dating back to the early 1990s, when trials were conducted by some American universities (Au & Lee ...
["Leading Virtual Reality in Education" OR "Leading Augmented Reality in Education"] Refined by: • Leading publications from top 5 countries: USA, People's Republic of China, Germany, England, and Italy ... The findings revealed that most papers during the era have concentrated on topics related to mobile learning and online ...
The adoption of immersive virtual reality (I-VR) as a pedagogical method in education has challenged the conceptual definition of what constitutes a learning environment. High fidelity graphics and immersive content using head-mounted-displays (HMD) have allowed students to explore complex subjects in a way that traditional teaching methods cannot. Despite this, research focusing on learning ...
The benefits of virtual reality in education go beyond academics as well to include cultural competence, the ability to understand another person's culture and values—an important skill in today's interconnected, global society. For example, a virtual reality field trip to other parts of the world, whether it be Peru or China, exposes ...
We conducted a meta-analysis on the impact of Virtual Reality (VR) in K-6 education. • Twenty-one journal papers published from 2010 to 2020 were coded and analysed. • Virtual reality has a medium-large positive effect on students' learning gains. • Immersive virtual reality promotes larger gains than semi and non-immersive systems. •
This paper provides a detailed analysis of the studies on VR applications in the realm of education. In this paper the last 8 years of data has been analyzed through bibliography research method, which depicts that the use of Virtual Reality (VR) in education has been significantly increased and the results show the growth of students in their academic key areas. This paper demonstrates the ...
In the field of education, virtual reality (VR) offers learners an immersive and interactive learning experience, allowing them to comprehend challenging concepts and ideas more efficiently and ...
This study is a systematic review of 20 years of research on the usage of virtual reality (VR) in K-12 and higher education settings, which aims to consolidate, evaluate, and communicate evidence that can inform both the theory and practice of VR-based instruction.
Virtual Reality (VR) and educational games are emerging technologies mediating a rapid transformation in the educational world. However, few studies have systematically analyzed Educational Virtual Reality Games (EVRGs) and how they have been applied in educational settings. This study reviewed 31 articles published in high impact journals and educational conference proceedings to unravel the ...
Findings show that VR improves learning outcomes when aligned with theories such as constructivism, experiential learning, and collaborative learning, enhancing learning outcomes across educational settings. Immersive technology, especially virtual reality (VR), transforms education. It offers immersive and interactive learning experiences. This study presents a systematic review focusing on ...
Summary. Virtual reality (VR) can be used to enhance student learning and engagement. Virtual reality-based education has the ability to completely transform the way educational content is delivered in this extremely digitalized world. The concept of virtual reality works on the premise of creating a virtual world, real or imagined, and allows ...
The chapter further discusses the reach of VR, i.e., how it is forming new perspectives for students in education. Most importantly, it will discuss the effects of virtual reality on student behavior, i.e., how this immersive technology is shaping their thought process, mindset and behavior, including the end result of using this tool of ...
Virtual Reality and Education Sandra Helsel Introduction As a profession, education is responding powerfully to the notion of virtual reality curriculum. Educators seem to have an instant - ... Dimensions of Virtual Realities In a landmark essay entitled "Informating with Virtual Reality," Spring set forth an important
a simulation generated by a computer, about a real or just an imaginary world. Another definition, more specific is: virtual reality is a computer interface that permits the user to interact in real time, in a tridimensional space generated by a computer, using their feelings, through special devices (Kimer, 2012).
Feature papers represent the most advanced research with significant potential for high impact in the field. ... in enhancing Learning Experiences (LX) in a more targeted, personalized, and engaging context. Specifically, Virtual Reality (VR) and Augmented Reality (AR) applications have the potential to enhance learning by offering students ...
Papers were selected with the goal of providing sufficient coverage of the topic by presenting an overarching summary rather than an exhaustive review of every type of application within VR. ... Springer; Cham, Switzerland: 2016. Shifting Virtual Reality Education to the Next Level—Experiencing Remote Laboratories Through Mixed Reality; pp ...
The use of Augmented Reality (AR) and Virtual Reality (VR) in education has been on the rise in recent years and provides a wealth of opportunities to leverage technology-enhanced learning (Tan et al., 2022). ... Number of papers in these three years is more than papers since 2011 to 2019. Precisely, we could confirm H 2 partially, ...
Virtual reality is a computer-generated scenario that gives the learner the immersive illusion of being somewhere else. It enables users to interact with a 3D environment without staring at a screen in front of them, by using electronic devices that send and receive information, such as activity sensors and motion trackers.
The name virtual reality comes from the definition of each of those words, "virtual" and "reality". The definition of "virtual" is 'near' and for "reality" is what a human being experiences (like in everyday). So, the term "virtual reality" basically means 'near-reality'.
If you are looking for inspiration for your next essay on virtual reality, here are 107 topic ideas and examples to get you started: The history and evolution of virtual reality technology; The impact of VR on the gaming industry; Virtual reality as a tool for education and training; The use of VR in healthcare for medical simulations and therapy
The main purpose of this article is to describe and summarize experiments with creating training applications using virtual reality devices. The arrival of new and more advanced virtual reality headsets opens up more opportunities and applications. This article deals with the current state of virtual reality and its application in education. The first chapter describes basic information about ...
Virtual reality (VR) is a rapidly evolving technology increasingly used in education. This research presents a bibliometric review and analysis of the research on VR in education in emerging economies, using data from the Scopus database. ... Top organizations based on several VR and AR papers are in medical education. The results of this ...